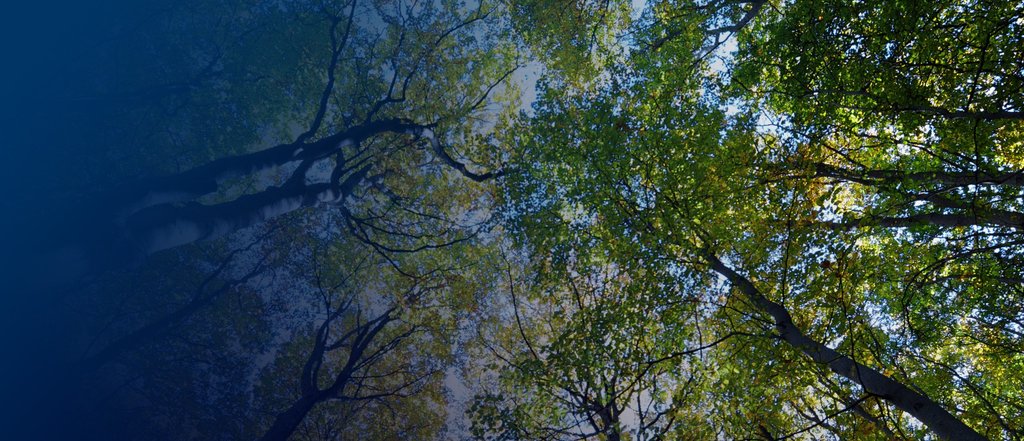
Ecosystems
The signal from radars that operate in frequency bands ranging from L band (with wavelengths around 20 cm) up to Ka band (with wavelengths shorter than 1 cm) can be used to characterize the surface and the vegetation by realistically modeling the interactions between the radar wave with both the vegetation and the surface. Longer wavelengths penetrate deeper than shorter ones and accumulate volume-dependent signatures in both the amplitude and phase of the received signals that can be used to reconstruct the properties of the vegetation and of the underlying soil.
These radar observations that probe vegetated areas with reasonably high spatial resolution (on the order of meters) hold the promise of revealing the 3-dimensional properties of the vegetation and their evolution in time. In order to produce these coherent radar observations, one must either have a single radar with multiple passes over a target area (taking many days for spaceborne systems) or have multiple platforms measuring the scattered signal, i.e. multistatic radars.
These multistatic radar observations strategies must be carefully designed such that the scientific goals of 3D vegetation structure and/or surface topography are met whilst consideration for the practical systems engineering requirements (power, antenna size, orbital, etc.) are met. In addition, because this requires the coherent interpretation of the coincident observations of several radars simultaneously, we need to improve the realism of our forward simulations in representing the different radar signatures of the vegetation and of the underlying surface and soil. Modeling the bistatic scattering of signals from various vegetation types from L band up to Ka band is critical to increasing the fidelity of our future mission designs.
Ecosystem Disturbances
In the Radar Science group, we also develop tools to help monitor forests and other vegetated areas through spaceborne and airborne repeat-pass SAR. We can detect fires, logging, or urban development by tracking scattering mechanisms over time.
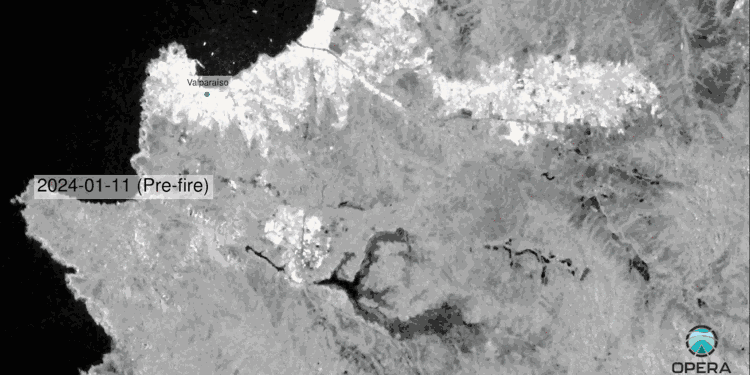
Animation shows OPERA Radiometric Terrain Corrected SAR backscatter Sentinel-1 VH images before, during, and after the fires near Valparaíso. Burn scars can be seen as light gray areas become darker indicating a decrease in volumetric scattering; the SAR fire map shows burn scars in red.
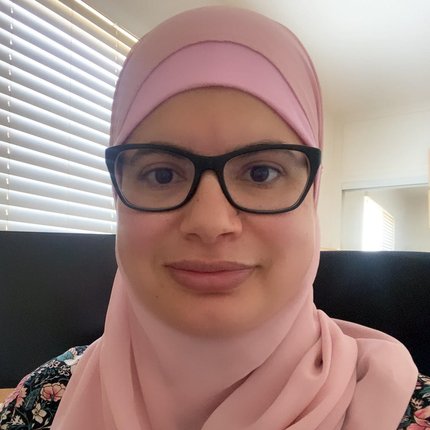
Ines Fenni
Ines Fenni received the M.Sc. degree in electrical engineering from ESIEE Paris, Noisy-le-Grand, France, in 2010, the M.Sc. degree in electrical engineering from Université de Bretagne Occidentale, Brest, France, in 2011, and the Ph.D. degree in electromagnetics from Université Pierre and Marie Curie (now Sorbonne University), Paris, France, in 2014. She joined the Jet Propulsion Laboratory (JPL), Pasadena, CA, USA, in 2015. Her current research interests include numerical methods and computational electromagnetics applied to the modeling of large scattering problems for Earth and planetary remote sensing.
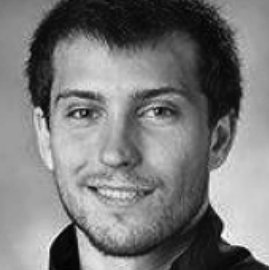
Eric Loria
Eric Loria received the bachelor’s degree in electrical engineering from Valparaiso University, Valparaiso, IN, USA in 2015 and the M.S. and Ph.D. degrees in electrical and computer engineering from the Ohio State University, Columbus, OH, USA in 2020. He is currently a signal analysis engineer at the Jet Propulsion Laboratory/Caltech, which he joined in 2020. His research interests include SAR and GNSS Reflectometry with an emphasis on electromagnetic scattering modeling, reflection signal processing methods for satellite platforms, and distributed radar systems architectures.
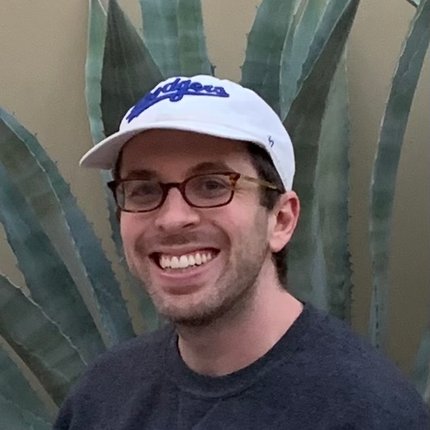
Charlie Marshak
Dr. Charlie Marshak joined JPL as a postdoc in 2017 and then as a signal analysis engineer in 2020. He earned his PhD in applied mathematics from UCLA in 2017, under the supervision of Andrea Bertozzi and Mason Porter. At JPL, he collaborated with Marc Simard to identify forest disturbances, estimate biomass, and monitor mangroves. Currently, he is a member of the Project Science team for OPERA and supports the generation of ARIA’s Geocoded Unwrapped Interferogram. Charlie is passionate about leveraging machine learning and signal processing to enhance the analysis of SAR and InSAR imagery. He also enjoys supporting NASA’s open-science vision via open-source python projects.
CL#24-5887