Lesson .
.Modeling the Orbits of Planets
Overview
In this activity, students explore the relationship between the masses of objects and the orbits they follow. They will make predictions and try to model different orbital scenarios using a gravity well model constructed in class.
Materials
- Hula hoop, 30” diameter or larger preferred
- Spandex OR other stretchable fabric that can be pulled tightly over the hoop
- 8-12 binder clips (large enough to clamp around the hoop ring and secure the fabric)
- Spheres of various sizes and masses (e.g., large metal spheres, glass or plastic marbles, wooden marbles, etc.)
Management
In advance of the lesson, create the gravity well model(s) as follows – making either one model for a class demonstration or one model per group of four to five students:
- Drape the fabric over the hula hoop and secure with 8-12 evenly spaced binder clips. A larger hoop can be made using lengths of flexible piping such as thin-walled, small-diameter PVC pipe. Be aware of the tension that builds up when the pipe is bent and be sure the ends are tightly secured when bending it into a hoop. Important safety note: Unsecured pipe can cause injury if its tension is released.
- Adjust the fabric and clips as needed so that the fabric is taut but has some give when a spherical mass is placed in the middle.
- Balance the model on the backs of three to four chairs to provide a stable, level support. Consider placing the chairs so the seats face inward and aren’t in the way when students gather around the model.
Background
Gravity is a force that affects everything in the universe. For example, it’s what holds us firmly on Earth’s surface and prevents us from floating away. But it’s so commonplace that we often don’t think about it until something falls.
Every object that has mass has gravity. The more massive an object is, the more gravity it has. A very massive object like Earth has a lot of gravity, but even a comparatively small human has gravity. So while Earth’s gravity pulls on us, our personal gravity also pulls on Earth. It’s such a small amount of gravity that there isn’t a noticeable effect on Earth.
Gravity is also the force that keeps natural satellites like the Moon and artificial satellites like the Mars Reconnaissance Orbiter in orbit. It also keeps planets orbiting our Sun.
Learn how NASA investigated a prediction from Einstein's theory of gravity, confirming the existence of a space-time vortex around Earth.
Albert Einstein, in his theory of general relativity, postulated that what we think of as empty space is actually three-dimensional space-time, a sort of fabric that fills the volume of the universe.
An object will warp space-time similar to the way a bowling ball warps the fabric of a trampoline, creating what’s known as a gravity well. The more massive an object is, the more warping of space-time occurs and the deeper the gravity well becomes.
Depending on its mass and velocity, an object entering another object’s gravity well could do several things.
- It could impact the other object if it is moving too slowly or its motion follows a path that intersects with the object.
- If it isn't moving too slowly and isn't on a path that intersects with the object, it could enter into an orbit around that object. Since both objects have a gravitational force, the two objects would actually orbit around a common center of mass. For example, the Earth and Moon orbit a common center of mass. But because the center of mass lies within Earth as a result of the planet’s much higher mass, it’s simpler to say that the Moon orbits Earth.
- If an object's velocity is high enough, it could pass by the second object and exit its gravity well, having its path altered by the curvature of space-time. Similarly, spacecraft need a high enough velocity to enter Earth’s orbit and not fall back down to the surface. Spacecraft need an even higher velocity to exit Earth’s gravity well and reach destinations like Mars. And they need a velocity that is even higher still to exit the Sun’s gravity well and leave the solar system.
Watch this video about how we launch things into space.
This activity explores some of those scenarios in a two-dimensional space. Keep in mind that in three dimensions, the warping occurs in all directions, so an object can enter, orbit, or exit a gravity well along any plane, depending on its speed and direction.
Procedures
Show students the gravity well model (see Management for assembly instructions) and explain that it is a two-dimensional representation of space-time.
Ask students to predict what will happen when you place a massive sphere weighing approximately 0.5 kg (1 lb) in the center.
With a lower-mass sphere representing a planet, ask students to predict what will happen if you release the sphere at the edge of the hoop. Have students explain how they came up with their prediction.
After releasing the sphere, ask students to explain why planets in the solar system don’t crash into the Sun. If students don’t mention the motion of planets, guide them to the fact that orbits are a combination of motions. Objects in orbit have motion toward the Sun, as pulled by gravity, and forward momentum that is perpendicular to the Sun’s gravitational pull.
Demonstrate pushing the smaller sphere perpendicular to the path taken by the previous sphere to create an orbit around the central mass.
Credit: NASA/JPL-CaltechA small sphere is pushed into orbit around the central mass.
Either as a class or in groups of four to five (with a hoop for each group), have students attempt the following orbits:
- Push a small mass into orbit around the larger central mass (as demonstrated in Step 6).
- Push two similarly sized masses into orbit around the larger central mass.
Credit: NASA/JPL-CaltechTwo small spheres are pushed into orbit around the central mass.
- Push a small mass orbiting a slightly larger mass into orbit around the large central mass.
Credit: NASA/JPL-CaltechA small sphere orbiting another slightly larger sphere is pushed into orbit around the central mass.
- Push a sphere with an equal mass to the central one into orbit around the central mass.
Credit: NASA/JPL-CaltechA sphere with an equal mass to the central one is pushed into orbit around the central mass.
Students should draw the paths made in their orbit scenarios on a sheet of paper. Students can use mobile devices, if available, to record videos at regular speed and in slow motion to capture the motion of the masses.
Have students attempt the following variations:
- Change the orbital path from circular to more elliptical.
Credit: NASA/JPL-CaltechA sphere is pushed into a more elliptical orbit around the central mass.
- Maximize the number of orbits made before the second object collides with the center mass.
Discussion
- How would the gravity well change if an object with a higher or lower mass were placed in the middle? How would that change the speed needed to orbit or exit a gravity well?
- What would this model look like if it were representing a black hole’s gravity well?
- Ask students to describe some limitations of the model they have been using. They should mention some of the following:
- The two-dimensional nature of the model means all objects orbit on the same plane, unlike the solar system in which objects orbit the Sun on different planes.
- The friction created by the fabric means orbiting objects slow down and fall in toward the central mass.
- What would the model look like in three dimensions? There would be warped planes in every direction on which the masses could orbit the central mass.
- The two-dimensional nature of the model means all objects orbit on the same plane, unlike the solar system in which objects orbit the Sun on different planes.
- The friction created by the fabric means orbiting objects slow down and fall in toward the central mass.
Assessment
- Students should accurately draw the paths of their orbit simulations.
- Students should be able to express that higher-mass objects create deeper gravity wells that require greater speeds to orbit or exit the well. Conversely, gravity wells with lower-mass objects at the center require lower speeds to orbit or exit the well.
Extensions
This model can be used to model how light is influenced by gravity when it travels through space. The phenomenon of light following a curved path around a gravity well is known as gravitational lensing, and it allows scientists to observe objects that might otherwise be hidden behind another object from our perspective. The amount of curvature can also tell scientists about the mass of the foreground object.
Several examples of gravitational lensing can be seen in this image from NASA’s James Webb Space Telescope of galaxy cluster SMACS 0723. See for example, the warped-looking galaxy to the upper right of the bright star near the center of the image. Learn more in this slideshow for students. Image credits: NASA, ESA, CSA, STScI | › Full image and caption.
- With a central mass on the fabric, ask students to predict the path of a lower-mass sphere if it is pushed past the central mass rather than pushed into an orbit around it. Demonstrate the path by pushing the sphere so that it passes near the central mass and travels toward the edge of the hoop.
- Have students mark a spot on the edge of the hoop with a sticky note to represent a telescope on Earth or in orbit. From the spot on the edge opposite the sticky note, have them use a smaller-mass sphere to simulate a photon of light passing nearby a large mass such as a planet, star, or even a galaxy to reach the telescope.
- Ask students to find multiple paths around the central mass to reach the telescope. They may find wider paths or paths on both sides of the central mass.
- Ask students how light that is curved along one or more of these paths might appear in an image captured by a telescope.
- Ask students how they think the light is affected by gravity if it doesn’t have mass.
A sphere simulates a photon of light passing nearby a central mass as observed by a telescope on Earth or in space.
About the Authors
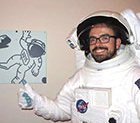
Lyle Tavernier
Educational Technology Specialist, NASA-JPL Education Office
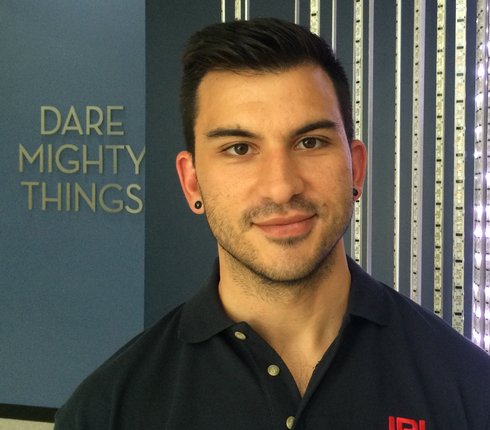
Brandon Rodriguez
Educator Professional Development Specialist, NASA-JPL Education Office
Lesson Last Updated: June 17, 2025