Lesson .
.The Air Up There: Making Space Breathable
Overview
In this activity, students will:
- Design an air-recycling system capable of capturing simulated carbon dioxide so astronauts can breath safely while on the International Space Station or a future Mars mission.
- Build and test a filter capable of "scrubbing" carbon dioxide out of the atmosphere while allowing air to flow.
- Calculate the efficacy of the filter.
- Use stoichiometry to determine how many filters are needed for a prolonged, human space mission.
Materials
- 2-3 index cards
- Tape
- Scissors
- Cotton balls
- Tissue paper
- Pipe cleaners
- Scale
- Black pepper, cocoa powder OR another small particulate
- 1-2 shoe boxes for the class
- Hairdryer or personal fan (airflow source)
- Student Worksheet – download PDF
- Instructor Guide – download PDF
Management
The testing apparatus can be constructed in advance:
- Cut a hole in one end of the box that allows air to flow in from the hairdryer or fan.
- On the opposite end, cut out a large section and attach a piece of tissue paper over the hole to demonstrate successful airflow.
- Having one or two of these boxes prepared allows students to test their filters when they are ready and cuts down on resource needs.
Emphasize that though this activity demonstrates a physical separation of chemicals, the carbon-dioxide devices aboard spacecraft are actually chemical filters.
Background
Whether they’re aboard the International Space Station or on a future mission to Mars, astronauts require systems that can create breathable air from their harsh surroundings. And chemistry plays an important role:
Currently, the International Space Station uses an absorption method to remove carbon dioxide (CO2) from the air. The absorption is accomplished in a chemical reaction using a sorbent called lithium hydroxide (LiOH). This method relies on the exothermic reaction of lithium hydroxide with carbon dioxide to create lithium carbonate (Li2CO3)(s) and water (H2O). Lithium hydroxide is an attractive choice for space flight because of its high absorption capacity for carbon dioxide and the small amount of heat produced by the reaction.
But when it comes to a future human mission to Mars, things get a bit more complicated. On the ISS, when filtration canisters are used up, we can send more on supply rockets. But on Mars, we can’t easily resupply the LiOH canisters. That means we need technology capable of producing breathable air over a longer time period.
One technique under consideration for Mars is to use chemistry and catalysis to transform the Red Planet’s harsh and unbreathable carbon dioxide atmosphere directly into oxygen. To test this technique, NASA will send a small instrument called Mars Oxygen In Situ Resource Utilization Experiment, or MOxIE, to the Red Planet in 2020 aboard the Mars 2020 rover. By the 2030s, it's possible NASA could send a larger version of MOxIE to Mars, and allow the system to run and create a safe, habitable area before astronauts even arrive. If successful, this technique will allow us to use an abundant resource on Mars to create a breathable environment for astronauts.
Procedures
In this activity, the class has been tasked by NASA to develop a device to recycle carbon dioxide into oxygen. This will allow a group of astronauts heading to Mars to survive in an atmosphere that would be otherwise inhospitable. The device, built by student engineers, should be able to capture simulated toxic carbon dioxide molecules represented by pepper, cocoa powder, etc. while allowing air to flow through to the other side of the device.
- Divide students into teams of three to four and introduce the challenge: Use the available materials to create a filter cartridge capable of capturing simulated toxic carbon dioxide (represented by pepper, cocoa powder or another particulate).
- Provide teams with a list of the materials available for constructing their filter cartridges. Index cards or pipe cleaners can be used to construct the perimeter or bracing of the filter, while other materials can be used to capture the chemical contaminants.
- Instruct teams that their filter cartridges:
- Must fit standing in the shoebox.
- Cannot completely impede airflow.
- Must be built with only the materials provided.
- Can be tested several times before final implementation.
- Have students draw out a design or blueprint before beginning construction.
- Have teams weigh their filter cartridges before testing them and record the pre-test weight on the Student Worksheet.
- Test the filter cartridges by placing them in the shoebox and using the fan or hairdryer to blow the particulate across them. A successful trial will capture the particulate in the filter cartridge while maintaining airflow at the exit.
- Once the test is complete, have teams re-weigh their filter cartridge and record the post-test weight on the Student Worksheet.
- Have teams calculate the amount (or mass) of simulated carbon dioxide captured by their filter cartridge by subtracting its pre-test weight from its post-test weight. For this experiment, every gram of solid material captured by the filter is equal to a gram of carbon dioxide.
- How did the filters perform? Discuss how much carbon dioxide teams captured for recycle and how well they maintained airflow. How well does this bode for our astronauts in terms of the amount and rate of reaction from CO2 to O2?
- Have teams revise one aspect of their designs to better capture the carbon dioxide. For example, did all of the powder make it to the filter or was it blown around the shoebox? Would changing the angle of airflow capture more CO2?
Discussion
- What did groups do to make an effective filter? What changes could have been made?
- How do these physical filtration techniques vary from the chemical filtration techniques used in space?
Assessment
Have students answer the following questions from the Student Worksheet. (Check their answers on the Instructor Guide.):
On the International Space Station, a device called the Contaminant Control Cartridge, which contains lithium hydroxide (LiOH), removes carbon dioxide (CO2) from the air. This process is represented by the following equation:
2 LiOH(s) + CO2(g) → Li2CO3(s) + H2O(g)
- Using the mass of carbon dioxide captured by your filter, determine how much lithium hydroxide each of your filter cartridges would need in order to effectively produce oxygen.
- A typical crew consists of six individuals and each Contaminant Control Cartridge contains 750 g of LiOH. Assuming that each crew member expels 42.0 g of CO2 per hour on average and that a mission is scheduled to last 18 days, how many cartridges must be carried on board the station?
On Mars, a device called the Mars Oxygen ISRU Experiment, or MOxIE, could convert the toxic carbon dioxide atmosphere to oxygen and vent out carbon monoxide in order to provide a breathable atmosphere for astronauts upon arrival. Balance the equation below and answer the following questions:
CO2(g) → O2(g) + CO(g)
- How many grams of oxygen would be produced by 1 kg of carbon dioxide? How many grams of carbon monoxide?
- Presently, MOXIE is capable of producing oxygen at a rate of 12 g per hour. If the astronauts require 30 kg of oxygen per month, how many days would MOXIE have to run in order to supply one month’s worth of oxygen?
- The byproduct of MOXIE, carbon monoxide, is also very poisonous. Discuss with your group how you could design a system to be sure CO is kept away from the astronauts and handled safely.
Extensions
Another stoichiometry issue facing astronauts on prolonged missions is loss of bone density. On Earth, we lose roughly 1% of bone mass (calcium carbonate) every year, yet astronauts lose 1-2% every month! One theory is this is due to buildup of sulfuric acid in our blood, arriving via amino acids obtained from animal protein.
- Write a complete balanced equation for the reaction between sulfuric acid and calcium carbonate to form calcium sulfate, carbon dioxide and water.
- While in space, an astronaut loses approximately 200 mg of calcium carbonate per day. Calculate the mass of sulfuric acid used in this process.
- A visit to Mars is on the horizon, but the duration of time required for a Mars mission is a concern to NASA. Currently, astronauts spend an average of 6 months on an ISS mission. A trip to Mars, however, would require 18 months of round-trip travel and an extra two to six months of on-the-ground research. It is currently projected that an astronaut would lose 1.5% of his or her pre-flight bone mineral density per month while on a Mars mission. Assume that there are 1,500 g of calcium in an astronaut’s bones pre-flight. Predict the mass of calcium that would remain after one year of a Mars mission.
(Check answers on the Instructor Guide.)
About the Author
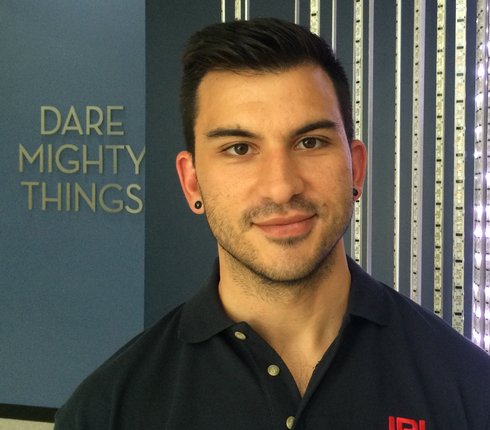
Brandon Rodriguez
Educator Professional Development Specialist, NASA-JPL Education Office
Lesson Last Updated: Sept. 25, 2024