Lesson .
.Marsbound! Mission to the Red Planet
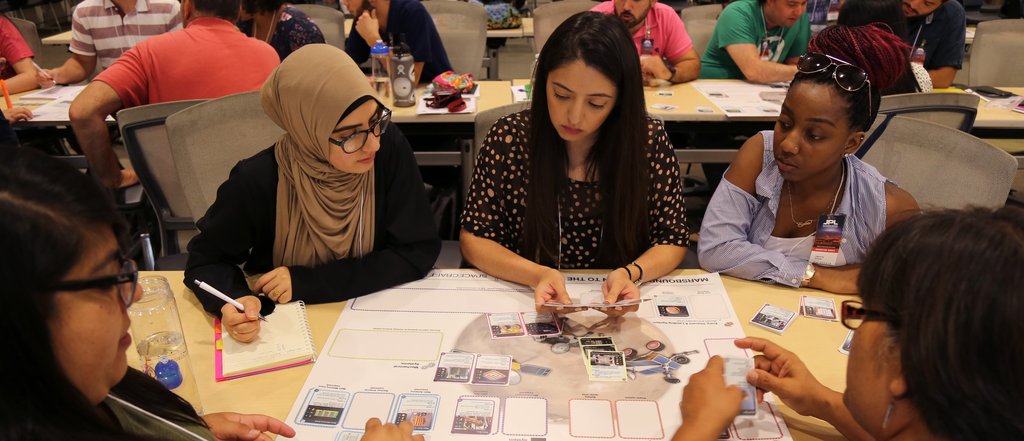
Educators play Marsbound during an educator professional development event at JPL.
Overview
Student teams creating Mars missions in the Marsbound activity will develop a science question that requires a technical design solution, and then they must design a solution. This means they will have to prioritize communication systems, computer systems, and scientific instrument packages while making trade-offs to remain within the limits of their mass, power and budget constraints. These are the same considerations made by teams at JPL developing actual missions to Mars.
Materials
- Equipment Cards (1 per team) – Download PDF
- Design Mat (1 per team) – 8.5x11 tiles PDF | large-format PDF
- Marsbound! Student Guide (1 per student) – Download PDF
- (Optional) Marsbound! Alignment Guide – Download PDF
- (Optional) Marsbound! Quick Reference Guide – Download PDF
- (Optional) Online edition – Download PPTX | Google Slides (Note: You will be prompted to open a copy of the Google Slides version, which will be saved to your Google Drive folder and can be edited as you see fit.)
- (Optional) Online edition - Download XLSX (Note: If unable to access the Spacecraft Design Log linked in the PowerPoint or Google Slides presentation, download this standalone spreadsheet file and open in Microsoft Excel or Google Sheets.)
Management
Tips for Remote Instruction
- For remote instruction, or to use a digital format of the game in class, download the PowerPoint (PPTX) or Google Slides from the materials list above. To play the online edition, give students access to the slides, where they can review cards, access the Spacecraft Design Log and plan their mission on the digital game mat.
- If you are prompted to request access, please speak with site or district technology personnel about accessing outside files. You can download the Spacecraft Design Log (XLSX) spreadsheet separately and open it in Microsoft Excel or Google Sheets.
Background
Show students these additional video and slideshow resources to make a connection to NASA’s Mars Rover “Curiosity.”
Vocabulary
- Criteria: A standard list of “rules” established so judgment or decisions are based on objective and defined ideas rather than subjective ones.
- Data: Facts, statistics, or information.
- Empirical Evidence: Knowledge gained through direct or indirect observation
- Engineering: A field in which humans solve problems that arise from a human need or desire by relying on their knowledge of science, technology, engineering design, and mathematics (derived from NRC Framework, 2012).
- Engineering Constraints: Limits placed on your mission by the hardware you use to accomplish the mission.
- Explanations: Logical descriptions applying scientific information
- Fly By: A spacecraft designed to go by a planet and study it on its way past
- Lander: a spacecraft designed to explore on the surface of a planet from a stationary position
- Mission: A spacecraft designed to explore space, seeking to answer scientific questions
- Models: A simulation that helps explain natural and human-made systems and shows possible flaws
- Observations: Specific details recorded to describe an objector phenomenon.
- Orbiter: A spacecraft designed to explore space, seeking to answer scientific questions
- Planet: A sphere moving in orbit around a star (e.g., Earth moving around our sun)
- Predict: A declaration about what will happen based on reason and knowledge
- Relationship: A connection between two objects
- Rocketry: A branch of science that deals with rockets and rocket propulsion.
- Rover: A robot designed to travel on the surface of a planet
- Scale: A comparative relation between objects such as size or distance
Procedures
Prepare the materials
Print out the Equipment Cards, Design Mat and Student Guide.
Tip: If you have printed the design mat and equipment cards from the website in black and white, ask your students to color the cards for you using a marker or colored pencil prior to laminating.
You can have students color the cards themselves using this table as a guide. Design Mat System Color Coordinating Card Numbers Launch System
Red
1-6
Power System
Orange
7-12
Science Instruments
Blue
13-25
Mobility System
Fuchsia
26-27
Mechanical System
Yellow
28-30
Entry, Descent, and Landing System
White
31-35
Computer System
Purple
36-38
Communications System
Aqua
39-41
Special Events
Green
42-47
You can have students color the cards themselves using this table as a guide.
Set Up the Scenario
Read the following:
Imagine that today, your school principal announces that you will be working on a new, very complex school project, a project that no one has ever done before. This project will be the single most important task you have ever been asked to complete thus far in your life. This project will be a group project, and you will be working with some people you know and others you don’t know. Everyone in your entire group will need to complete the group project successfully or no one will pass. In fact, the project is so important, you will be working on it in every one of your classes, during an afterschool program, and as homework. You will probably be working on it at least 12+ hours a day and during many weeks; you will work through the weekend, too! You will have just 2 years to complete the project. The project is so complex and difficult, that you will have to revise and rewrite the plans for the project constantly. When the project deadline arrives, the group will have to show the completed project to the school, principal and, oh yes, all the news stations in the world will be there as well. You will have no extensions on the deadline. No pressure, but everyone is counting on you!
NASA mission planners, engineers, and scientists go through much the same process when designing and building space missions to Mars and other destinations. Many times, they are faced with tasks that have never been tried before. Imagine that they have spent 2 years of their lives, 12+ hours a day, planning, building, planning, testing, retesting, re-planning, re-building, re-testing, packaging, shipping, unpacking, testing, and re-testing, all in an attempt to do everything in their power to ensure their mission makes it to the surface of Mars.Explain they will be participating in a simulation to design a mission to Mars. As part of “qualifying” for the mission planning, ask students to complete the Pre-Ideas Worksheet in the Student Guide. This survey will help to establish their current understanding of mission planning and engineering constraints. Students will use this information during the Post-Ideas part of the activity as part of their individual assessments.
Hand out the Marsbound! Student Guide, Equipment Cards and Design Mat.
Construct a Science Question
The purpose of this activity is to familiarize students with national goals for the exploration of Mars, and to enable students to categorize science questions according to these goals.
Discuss NASA’s four Mars Exploration Program goals and strategies with students.
NASA’s Mars Exploration Program Goals
- Determine if life ever arose on Mars. All life, as we know it, requires water to survive. In fact, on Earth we have found life wherever there is water, even in places we didn’t think life could exist, such as frozen deserts of Antarctica. Is the same thing true of Mars? Because of the low temperatures and thin atmosphere of Mars today, we know that there is currently no liquid water on the surface of the planet. But was that always true?
- Characterize the climate of Mars. If we can understand what the climate of Mars is like today and how it changes, we will have a better idea of what the climate of Mars was like in the past. The atmosphere of Mars is mostly carbon dioxide, but two other important components are water vapor and dust. With enough information, we can begin to create a picture of the overall climate of Mars now and what it may have once been like.
- Characterize the geology of Mars. Rocks and minerals on the surface of Mars can tell us a great deal about a planet’s past. By studying surface morphology and patterns and types of features found on the surface, we can find a permanent record of the history of Mars in its rocks.
- Prepare for human exploration. Humans are naturally curious. No robot will ever have the flexibility of a human explorer, so someday we will want to travel to Mars ourselves to study the planet and its history directly. Because of the difficulty and the number of challenges, robotic spacecraft must pave the way for humans to follow. One important task is to study new techniques for entering the Martian atmosphere and landing on the surface. We will also need to understand the dangers humans will face on the surface of Mars.
Working with their teams, have students categorize each question under each goal.
Tip: Keep in mind that a science question (mission objective) may apply to more than one science goal. There is no one "correct" answer; it is more important that your students can justify the reasons for the categorization.
Determine Science Goals
Student teams will discuss possible science objectives among themselves.
Tip: Space is provided for five science goals, but your students will be hard-pressed to design a spacecraft (under budget) that can meet all five goals. This constraint is intentional, as it will guide them to revise their mission plan by going all the way back to the original Mission Goals page. This iterative process happens quite often in the real world as well.
Students will also determine a technological solution by deciding whether they want to fly a lander, orbiter or fly-by mission to Mars.
Tip: In preparing students to make choices on whether to use a lander, orbiter, or fly-by, you can use the Strange New Planet lesson for a hands-on activity about exploring new planets.
Design a Technical Solution
Student teams will begin to design the actual spacecraft that they will use for their mission. To facilitate this, each typical system that could be onboard a spacecraft is presented on its own “trading card.” Students will need to read each card carefully, as the text provides clues about the uses and limitation for that particular piece of hardware.
Important! Hold the (Green) Special Events cards until the end of the simulation.
Students will begin the simulation by choosing a (Red) Rocket Card and Rocket Nose Cone (required). The rocket card will determine the Mass Limit for the mission and will include the Cost in millions of dollars. The nose cone will be additional Weight and money, so students will need to record this information into their (G) Spacecraft Design Log.
Students will then choose a (Orange) Power System Card. This card will determine the Power available during the mission.
From here, students will choose their (Purple) Computer Systems, (Aqua) Communication Systems, and (Blue) Science Instruments cards to achieve their science goals stated in Activity 2. These will help to increase Science Return.
If students have chosen a rover or lander for their mission, rovers will need to include a (Fuchsia) Mobility System, and both rovers and landers will require (White) Entry, Descent, & Landing Systems.
The final decision will be optional (Yellow) Mechanical Systems. These can increase the Science Return, but should be considered last due to budget constraints. Remind students to keep a tally in their (G) Spacecraft Design Log to ensure they are staying within budget, power and mass. *Tip: The teacher will need to define the budget. Lower amounts make it a more challenging activity, while higher amounts make it less challenging. Starting with $250 million is recommended as a good “average” level of difficulty for any of the missions.
When students have created a mission within budget, power, and mass, they can now select a (Green) Special Events card. Half of these cards are Spin-offs or advances in technology that can be commercialized. These add money to the budget. The other half of the cards is failures or cuts to the budget. These take away money from the budget. Allow students time to adjust their mission to accommodate these scenarios. *Tip: Ask students to use a pencil on their (G) Spacecraft Design Log so that they can easily erase when necessary.
The final step will be launch day. The Budget: Science Return ratio will establish the order of launch. For each mission, calculate the $/science ratio by dividing the amount of money spent on the mission by the number of science stars earned. The first place team with the lowest Science Return ratio and falling at or below budget, mass and power. Students will roll the die to determine if their mission launched successfully. The type of rocket they chose will determine the success rate. For example, the Heavy-Lift Rocket is high risk, only lifting off successfully 3 out of 6 times. If students roll a 1, 2, or 3, they lift successfully. If they roll a 4, 5, or 6, launch fails and the mission is over.
Analyze Constraints
Have students refer to worksheets J and K on the Marsbound Student Guide.
This activity focuses on the concept of engineering constraints and getting students to identify where they participated in the engineering design cycle throughout the lesson.
Encourage students to think of everything that limited what they attempted to do with their mission, how they tested ideas, and how the team solved problems.
Each of these examples should be written directly onto (K) Engineering Design Cycle, demonstrating the iterative engineering process they have just participated in.
Examples would include the limited mass that can be lifted by the rocket booster available, the electrical power that is required by each system onboard, and staying within the pre-determined budget including all of the group discourse and problem solving required to solve those engineering problems.
Tip: Ask students to consider other constraints that might limit a mission beyond what they discussed here. For example, a lander mission needs to be able to land safely in the terrain chosen to meet the science goals. After a little research, your students may realize that it is impossible to land safely in some kinds of terrain (such as mountains or the slopes of a volcano).
Ask students to share their constraints and accommodations with the class. The goal of this sharing process is to have the students listen critically to their peers’ explanations, explain their own solutions, and question others’ explanations. After class sharing, take a few minutes to discuss and reaffirm some of the items they may have mentioned and highlight those missed:
- Size and Mass: Some engineering constraints are due to the strength of the rocket you use to send your spacecraft to Mars. To send every instrument to Mars would require a rocket so large that it doesn’t even exist.
- Budget: The United States Congress sets the budget, the total amount of money available to spend for each NASA mission. NASA must therefore, design missions to achieve as many science goals as possible, while still staying within budget. Bigger rocket boosters can carry bigger spacecraft. Unfortunately, they cost a lot more to launch.
- Power: Every spacecraft needs power in order to function. The more instruments that are onboard, the more power is needed for them to operate. Solar panels must be very large, but even so, still do not produce a lot of power. They require a great deal of direct sunlight to operate, so missions with solar panels are limited to being near the Martian equator, and can only operate for about 3 months of the year. Fuel cells create power through a chemical reaction much like batteries and produce a moderate amount of power, but they will only function for a limited period of time, generally only a few days or weeks. Radioisotope power systems (RPS) produce power from the heat generated by decaying radioactive materials. They produce a lot of power and can operate at any time of year and anywhere on the surface. They are quite heavy, extremely expensive, and require more precautions.
- Reliability: Some rockets are more reliable than others.
- Bottom line: Engineering constraints often force you to make trade-offs. These constraints may keep you from being able to achieve all of your science goals, so you have to choose the equipment that will allow you to achieve as many of your science goals as possible.
Apply Skills to a Novel Problem
Choose one of the following:
- Ask students to trade their mission plan with another team. Each group will evaluate the mission teams science goal and objectives along with identifying any engineering constraints that might have been missed. Evaluation teams will make recommendations for improvement to the mission team.
- Rerun the simulation, planning for a sample-return mission from the surface of Mars to Earth with a budget of $500 million and valued at a science return of five. Teams will need to include:
- A rover with robotic arm for sample collection
- A launch vehicle to get the sample off of the planet or moon and into orbit
- An orbiter to catch the launched sample
- A launch vehicle to get from the orbiter to Earth
- Research possible landing sites to consider additional engineering constraints.
- Give student groups a copy of the Comparing Two Mars Rover Projects and ask them to reflect on the differences in the design of these Rover missions. What are some of the differences in engineering constraints that must have been overcome for each mission?
Assessment
Have students complete the post-ideas (worksheet J from the Marsbound! Student Guide). Students will need to refer back to the pre-survey and simulation to respond to these questions.
Extensions
- Choose another activity from "Analyze Constraints" step in the procedures section.
- Have students submit a formal written proposal (utilizing Common Core State Standards and Next Generation Science Standards Arguing from Evidence) competing for additional funding from their proposed mission. Proposal winnings will not be calculated in Budget : Science Ratio allowing the budget to remain a budget.
- Have students create a spreadsheet to keep track of calculations.
The concept for the online edition of this lesson was created by Guadalupe de la O at Alliance Renee and Meyer Luskin Academy in Los Angeles.
Lesson Last Updated: Nov. 4, 2024