Teachable Moments | May 12, 2022
Telescopes Get Extraordinary View of Milky Way's Black Hole
Find out how scientists captured the first image of Sagittarius A*, why it's important, and how to turn it into a learning opportunity for students.
Our home galaxy, the Milky Way, has a supermassive black hole at its center, but we’ve never actually seen it – until now. The Event Horizon Telescope, funded by the National Science Foundation, has released the first image of our galactic black hole, Sagittarius A* (pronounced “Sagittarius A-star” and abbreviated Sgr A*).
Read on to find out how the image was acquired and learn more about black holes and Sagittarius A*. Then, explore resources to engage learners in the exciting topic of black holes.
How Black Holes Work
A black hole is a location in space with a gravitational pull so strong that nothing, not even light, can escape it. A black hole’s outer edge, called its event horizon, defines the spherical boundary where the velocity needed to escape exceeds the speed of light. Matter and radiation fall in, but they can’t get out. Because not even light can escape, a black hole is literally black. Contrary to their name’s implication, black holes are not empty. In fact, a black hole contains a great amount of matter packed into a relatively small space. Black holes come in various sizes and can exist throughout space.
We can surmise a lot about the origin of black holes from their size. Scientists know how some types of black holes form, yet the formation of others is a mystery. There are three different types of black holes, categorized by their size: stellar-mass, intermediate-mass, and supermassive black holes.
Stellar-mass black holes are found throughout our Milky Way galaxy and have masses less than about 100 times that of our Sun. They comprise one of the possible endpoints of the lives of high-mass stars. Stars are fueled by the nuclear fusion of hydrogen, which forms helium and other elements deep in their interiors. The outflow of energy from the central regions of the star provides the pressure necessary to keep the star from collapsing under its own weight.
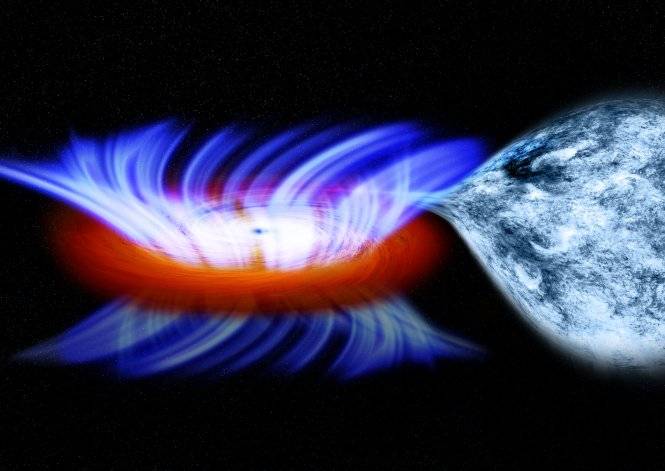
This illustration shows a binary system containing a stellar-mass black hole called IGR J17091-3624. The strong gravity of the black hole, on the left, is pulling gas away from a companion star on the right. This gas forms a disk of hot gas around the black hole, and the wind is driven off this disk. Image credit: NASA/CXC/M.Weiss | › Full image and caption
Once the fuel in the core of a high-mass star has completely burned out, the star collapses, sometimes producing a supernova explosion that releases an enormous amount of energy, detectable across the electromagnetic spectrum. If the star’s mass is more than about 25 times that of our Sun, a stellar-mass black hole can form.
Intermediate-mass black holes have masses between about 100 and 100,000 times that of our Sun. Until recently, the existence of intermediate-mass black holes had only been theorized. NASA’s Chandra X-ray Observatory has identified several intermediate-mass black hole candidates by observing X-rays emitted by the gas surrounding the black hole. The Laser Interferometer Gravitational Wave Observatory, or LIGO, funded by the National Science Foundation, detected the merger of two stellar-mass black holes with masses 65 and 85 times that of our Sun forming an intermediate-mass black hole of 142 solar masses. (Some of the mass was converted to energy and about nine solar masses were radiated away as gravitational waves.)
Supermassive black holes contain between a million and a billion times as much mass as a stellar-mass black hole. Scientists are uncertain how supermassive black holes form, but one theory is that they result from the combining of stellar-mass black holes.
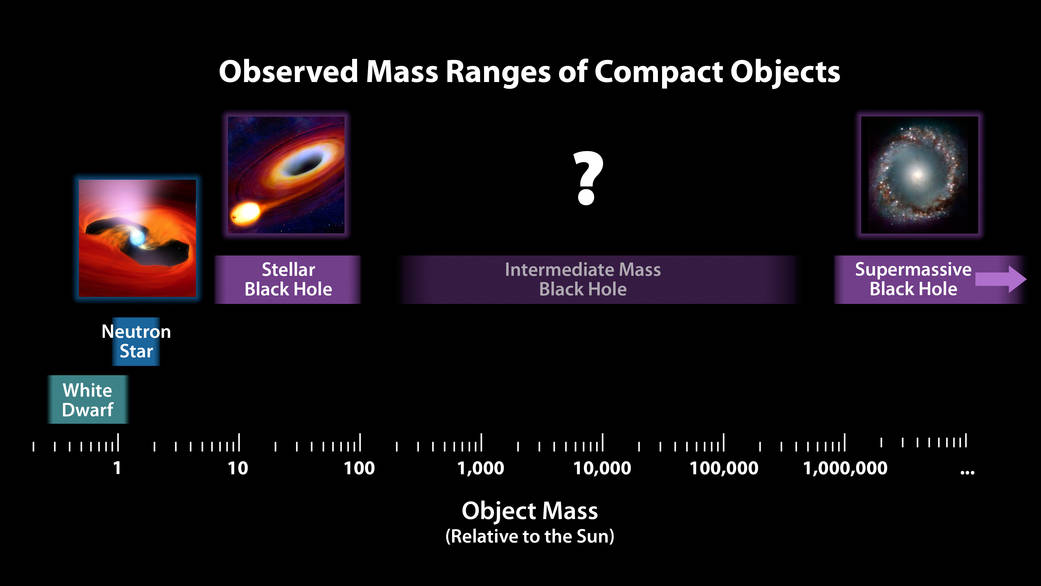
This chart illustrates the relative masses of super-dense cosmic objects, ranging from white dwarfs to the supermassive black holes encased in the cores of most galaxies. | › Full image and caption
Our local galactic center’s black hole, Sagittarius A*, is a supermassive black hole with a mass of about four million suns, which is fairly small for a supermassive black hole. NASA’s Hubble Space Telescope and other telescopes have determined that many galaxies have supermassive black holes at their center.
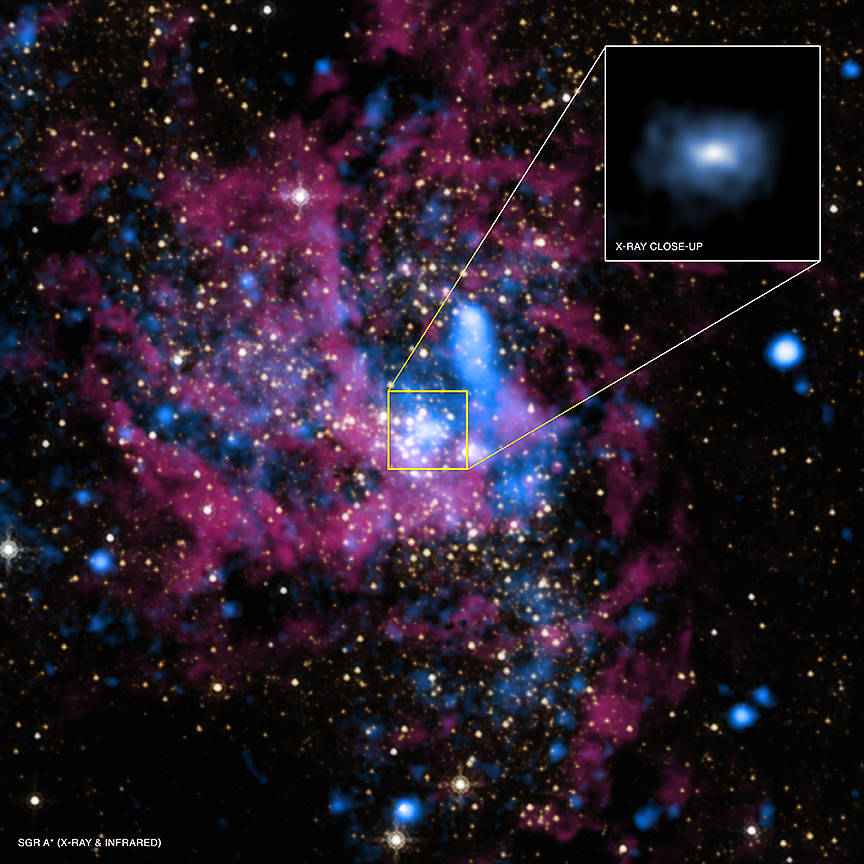
This image shows the center of the Milky Way galaxy along with a closer view of Sagittarius A*. It was made by combining X-ray images from NASA's Chandra X-ray Observatory (blue) and infrared images from the agency's Hubble Space Telescope (red and yellow). The inset shows Sgr A* in X-rays only, covering a region half a light year wide. Image credit: X-ray: NASA/UMass/D.Wang et al., IR: NASA/STScI | › Full image and caption
Why They're Important
Black holes hold allure for everyone from young children to professional astronomers. For astronomers, in particular, learning about Sagittarius A* is important because it provides insights into the formation of our galaxy and black holes themselves.
Understanding the physics of black hole formation and growth, as well as their surrounding environments, gives us a window into the evolution of galaxies. Though Sagittarius A* is more than 26,000 light years (152 quadrillion miles) away from Earth, it is our closest supermassive black hole. Its formation and physical processes influence our galaxy as galactic matter continually crosses the event horizon, growing the black hole’s mass.
Studying black holes also helps us further understand how space and time interact. As one gets closer to a black hole, the flow of time slows down compared with the flow of time far from the black hole. In fact, according to Einstein’s theory of general relativity, the flow of time slows near any massive object. But it takes an incredibly massive object, such as a black hole, to make an appreciable difference in the flow of time. There's still much to learn about what happens to time and space inside a black hole, so the more we study them, the more we can learn.
How Scientists Imaged Sagittarius A*
Black holes, though invisible to the human eye, can be detected by observing their effects on nearby space and matter. As a result of their enormous mass, black holes have extremely high gravity, which pulls in surrounding material at rapid speeds, causing this material to become very hot and emit X-rays.
This video explains how Sagittarius A* appears to still have the remnants of a blowtorch-like jet dating back several thousand years. Credit: NASA | Watch on YouTube
X-ray-detecting telescopes such as NASA’s Chandra X-ray Observatory can image the material spiraling into a black hole, revealing the black hole’s location. NASA’s Hubble Space Telescope can measure the speed of the gas and stars orbiting a point in space that may be a black hole. Scientists use these measurements of speed to determine the mass of the black hole. Hubble and Chandra are also able to image the effects of gravitational lensing, or the bending of light that results from the gravitational pull of black holes or other high-mass objects such as galaxies.

The thin blue bull's-eye patterns in this Hubble Space Telescope image are called "Einstein rings." The blobs are giant elliptical galaxies roughly 2 to 4 billion light-years away. And the bull's-eye patterns are created as the light from galaxies twice as far away is distorted into circular shapes by the gravity of the giant elliptical galaxies. | › Full image and caption
To directly image the matter surrounding a black hole, thus revealing the silhouette of the black hole itself, scientists from around the world collaborated to create the Event Horizon Telescope. The Event Horizon Telescope harnesses the combined power of numerous telescopes around the world that can detect radio-wave emissions from the sky to create a virtual telescope the size of Earth.
Narrated by Caltech’s Katie Bouman, this video explains how she and her fellow teammates at the Event Horizon Telescope project managed to take a picture of Sagittarius A* (Sgr A*), a beastly black hole lying 27,000 light-years away at the heart of our Milky Way galaxy. Credit: Caltech | Watch on YouTube
In 2019, the team released the first image of a black hole's silhouette when they captured the glowing gasses surrounding the M87* galactic black hole nearly 53 million light-years (318 quintillion miles) away from Earth. The team then announced that one of their next endeavors was to image Sagittarius A*.
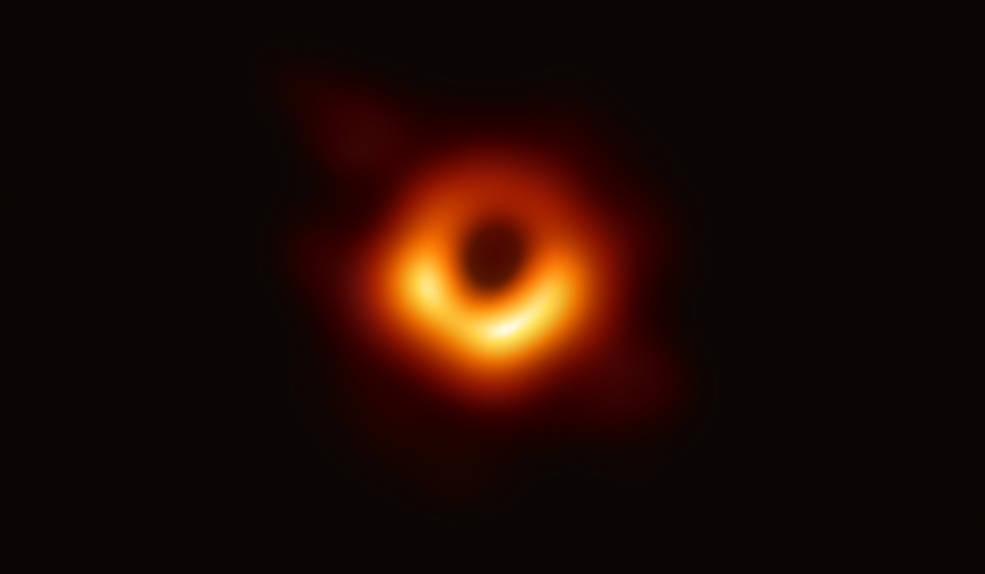
Captured by the Event Horizon Telescope in 2019, this image of the the glowing gasses surrounding the M87* black hole, was the first image ever captured of a black hole. Image credit: Event Horizon Telescope Collaboration | + Expand image
To make the newest observation, the Event Horizon Telescope focused its array of observing platforms on the center of the Milky Way. A telescope array is a group of telescopes arranged so that, as a set, they function similarly to one giant telescope. In addition to the telescopes used to acquire the M87* image, three additional radio telescopes joined the array to acquire the image of Sagittarius A*: the Greenland Telescope, the Kitt Peak 12-meter Telescope in Arizona, and the NOrthern Extended Millimeter Array, or NOEMA, in France.
This image of the center of our Milky Way galaxy representing an area roughly 400 light years across, has been translated into sound. Listen for the different instruments representing the data captured by the Chandra X-ray Observatory, Hubble Space Telescope, and Spitzer Space Telescope. The Hubble data outline energetic regions where stars are being born, while Spitzer's data captures glowing clouds of dust containing complex structures. X-rays from Chandra reveal gas heated to millions of degrees from stellar explosions and outflows from Sagittarius A*. Credit: Chandra X-ray Observatory | Watch on YouTube
The distance from the center of Sagittarius A* to its event horizon, a measurement known as the Schwarzschild radius, is enormous at seven million miles (12,000,000 kilometers or 0.08 astronomical units). But its apparent size when viewed from Earth is tiny because it is so far away. The apparent Schwarzschild radius for Sagittarius A* is 10 microarcseconds, about the angular size of a large blueberry on the Moon.
Acquiring a good image of a large object that appears tiny when viewed from Earth requires a telescope with extraordinarily fine resolution, or the ability to detect the smallest possible details in an image. The better the resolution, the better the image and the more detail the image will show. Even the best individual telescopes or array of telescopes at one location do not have a good enough resolution to image Sagittarius A*.
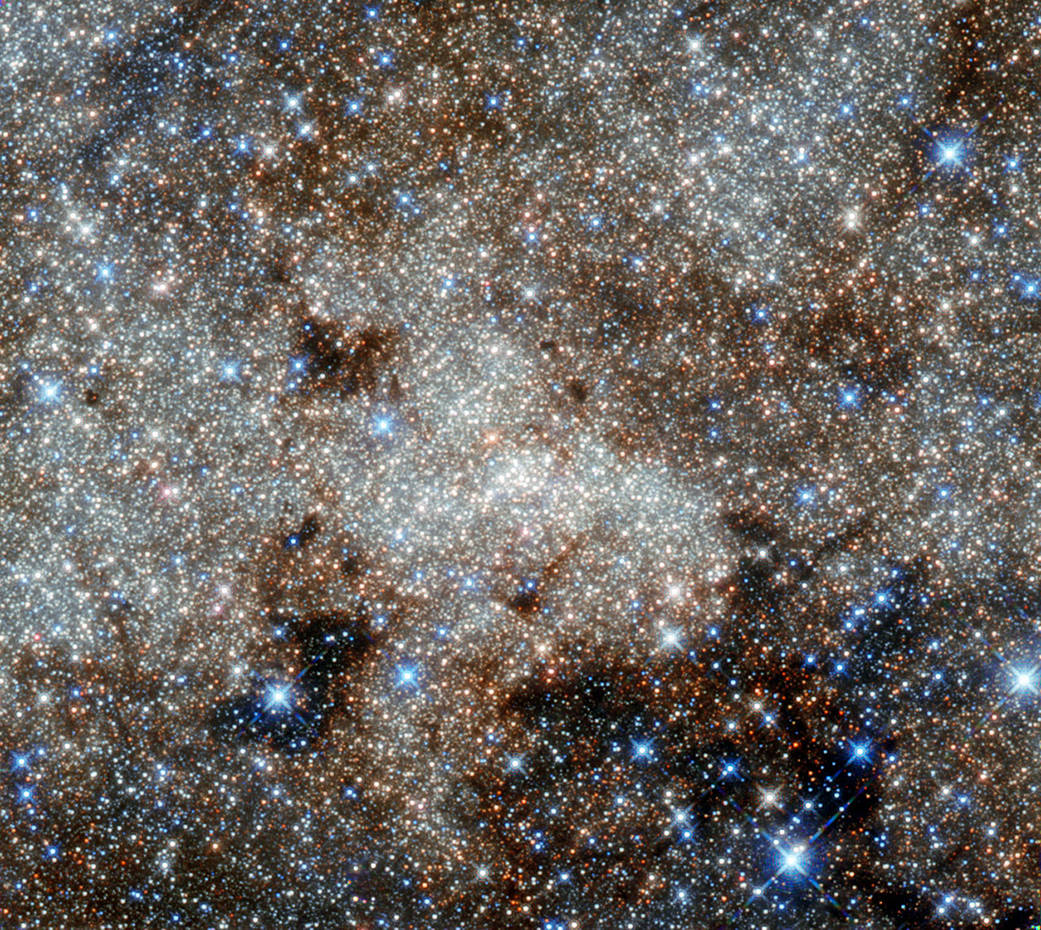
This image captured by NASA's Hubble Space Telescope shows the star-studded center of the Milky Way towards the constellation of Sagittarius. Even though you can't see our galaxy's central black hole directly, you might be able to pinpoint its location based on what you've learned about black holes thusfar. Image credit: NASA, ESA, and G. Brammer | › Full image and caption
The addition of the 12-meter Greenland Telescope, though a relatively small instrument, widened the diameter, or aperture, of the Event Horizon Telescope to nearly the diameter of Earth. And NOEMA – itself an array of twelve 15-meter antennas with maximum separation of 2,500 feet (760 meters) – helped further increase the Event Horizon Telescope’s light-gathering capacity.
Altogether, when combined into the mighty Event Horizon Telescope, the virtual array obtained an image of Sagittarius A* spanning about 50 microarcseconds, or about 1/13th of a billionth the span of the night sky.

Sagittarius A* is more than 26,000 light years (152 quadrillion miles) away from Earth and has the mass of 4 million suns. Image credit: Event Horizon Telescope | › Full image and caption
While the Event Horizon Telescope was busy capturing the stunning radio image of Sagittarius A*, an additional worldwide contingent of astronomical observatories was also focused on the black hole and the region surrounding it. The aim of the team, known as the Event Horizon Telescope Multiwavelength Science Working Group, was to observe the black hole in other parts of the electromagnetic spectrum beyond radio. As part of the effort, X-ray data were collected by NASA’s Chandra X-ray Observatory, Nuclear Spectroscopic Telescope (NuSTAR), and Neil Gehrels Swift Observatory, additional radio data were collected by the East Asian Very Long-Baseline Interferometer (VLBI) network and the Global 3 millimeter VLBI array, and infrared data were collected by the European Southern Observatory’s Very Large Telescope.
The data from these multiple platforms will allow scientists to continue building their understanding of the behavior of Sagittarius A* and to refine their models of black holes in general. The data collected from these multiwavelength observations are crucial to the study of black holes, such as the Chandra data revealing how quickly material falls in toward the disk of hot gas orbiting the black hole’s event horizon. Data such as these will hopefully help scientists better understand black hole accretion, or the process by which black holes grow.
Teach It
Check out these resources to bring the real-life STEM of black holes into your teaching, plus learn about opportunities to involve students in real astronomy research.
-
Educator Guide: Dropping In With Gravitational Waves
Students develop a model to represent the collision of two black holes, the gravitational waves that result and the waves' propagation through spacetime.
Subject Science
Grades 6-8
Time 30-60 mins
-
Teachable Moments: How Scientists Captured the First Image of a Black Hole
Find out how scientists created a virtual telescope as large as Earth itself to capture the first image of a black hole's silhouette.
-
Teachable Moments: Gravitational Waves Detected for the First Time
Find out how researchers proved part of Albert Einstein’s Theory of General Relativity, then create a model of the Nobel Prize-winning experiment in the classroom.
Explore More
Articles
-
Teachable Moments: Learn About the Universe With the James Webb Space Telescope
Get a look into the science and engineering behind the largest and most powerful space telescope ever built while exploring ways to engage learners in the mission.
-
JPL Education ‘Teachable Moment’ Inspires Winning Science Fair Project
A “teachable moment” turned into a science fair win for an eighth-grader in Ontario, Canada, who based his project on a classroom activity from NASA’s Jet Propulsion Laboratory.
Educator Guides
Student Activities
Check out these related resources for students from NASA’s Space Place
Across the NASA-Verse
- Educator Guide: Black Hole Math
- NASA/IPAC TeacherArchive Research Program
- Student Resources: Chandra
- Articles: Hubble - Black Holes
- Audio: Sonification of the Milky Way galactic center
- Audio: Sonification of the M87 black hole
- Interactive: Sagittarius A*
- Videos: Hubble - Black Holes
- Website: NASA Science - Black Holes
- Download: A Galaxy Full of Black Holes Presentation
- Expert Talk: Imaging a Black Hole Lecture
- Article: Black Hole Image Makes History
- Graphic: Anatomy of a Black Hole
This Teachable Moment was created in partnership with NASA’s Universe of Learning. Universe of Learning materials are based upon work supported by NASA under award number NNX16AC65A to the Space Telescope Science Institute, working in partnership with Caltech/IPAC, Center for Astrophysics | Harvard & Smithsonian, and the Jet Propulsion Laboratory.
TAGS: Black hole, Milky Way, galaxy, universe, stars, teachers, educators, lessons, Teachable Moments, K-12, science
Teachable Moments | March 6, 2020
We've Got the Formula for a Stellar Pi Day
Update: March 16, 2020 – The answers to the 2020 NASA Pi Day Challenge are here! View the illustrated answer key (also available as a text-only doc).
In the News
Our annual opportunity to indulge in a shared love of space exploration, mathematics and sweet treats has come around again! Pi Day is the March 14 holiday that celebrates the mathematical constant pi – the number that results from dividing any circle's circumference by its diameter.
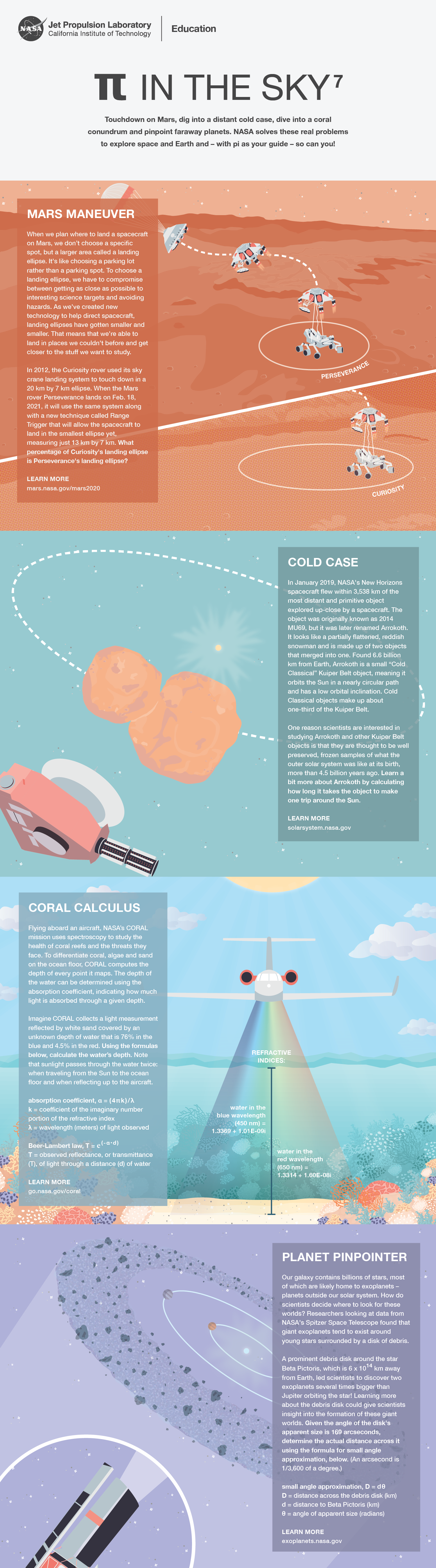
Visit the Pi in the Sky 7 lesson page to explore classroom resources and downloads for the 2019 NASA Pi Day Challenge. Image credit: NASA/JPL-Caltech | + Expand image

A new Mars landing technique called Range Trigger is reducing the size of the ellipse where spacecraft touch down. Image credit: NASA/JPL-Caltech | › Full image and caption
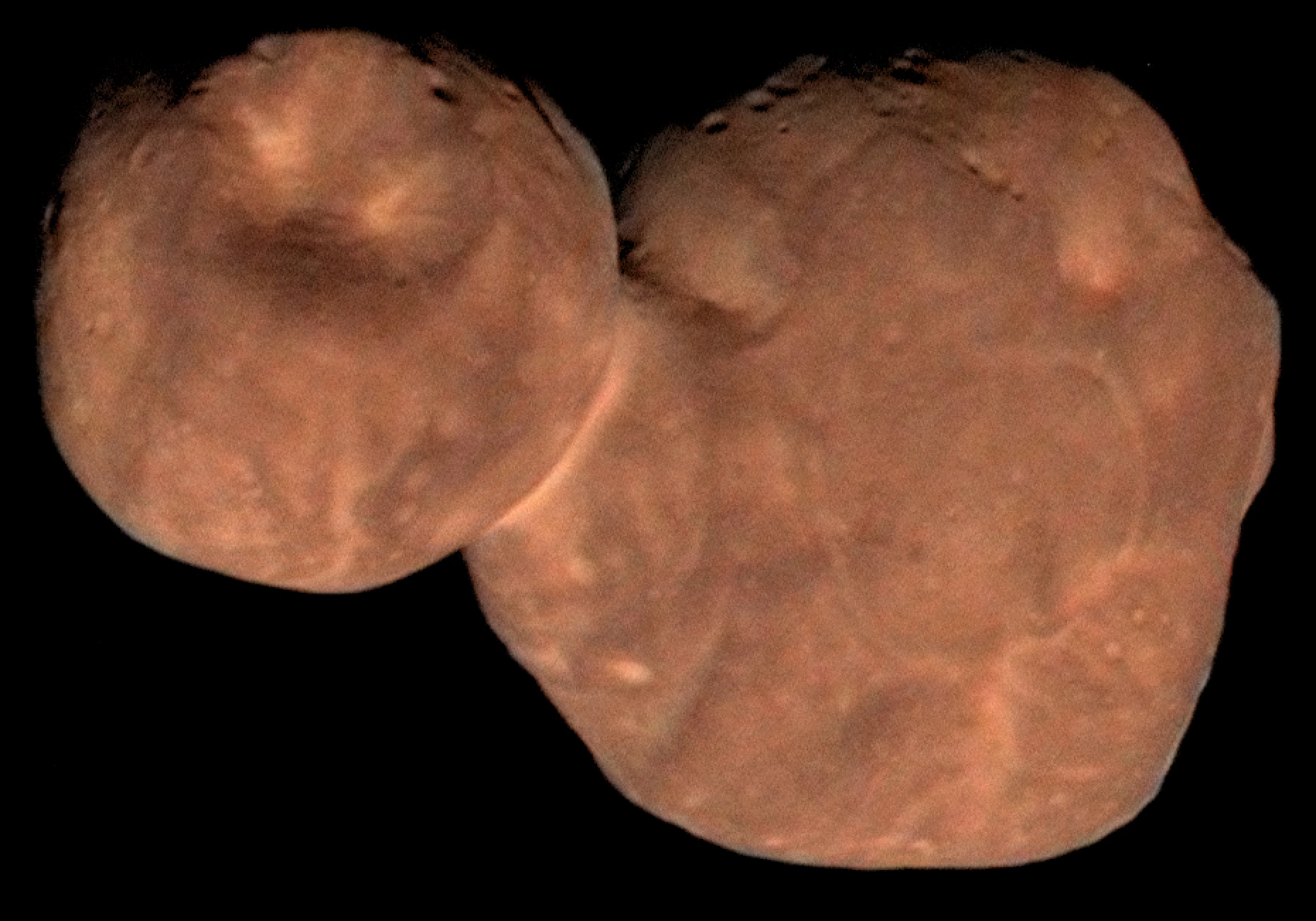
Composite image of the Kuiper Belt object Arrokoth from NASA's New Horizons spacecraft. Image credit: NASA/Johns Hopkins University Applied Physics Laboratory/Southwest Research Institute/Roman Tkachenko | › Full image and caption
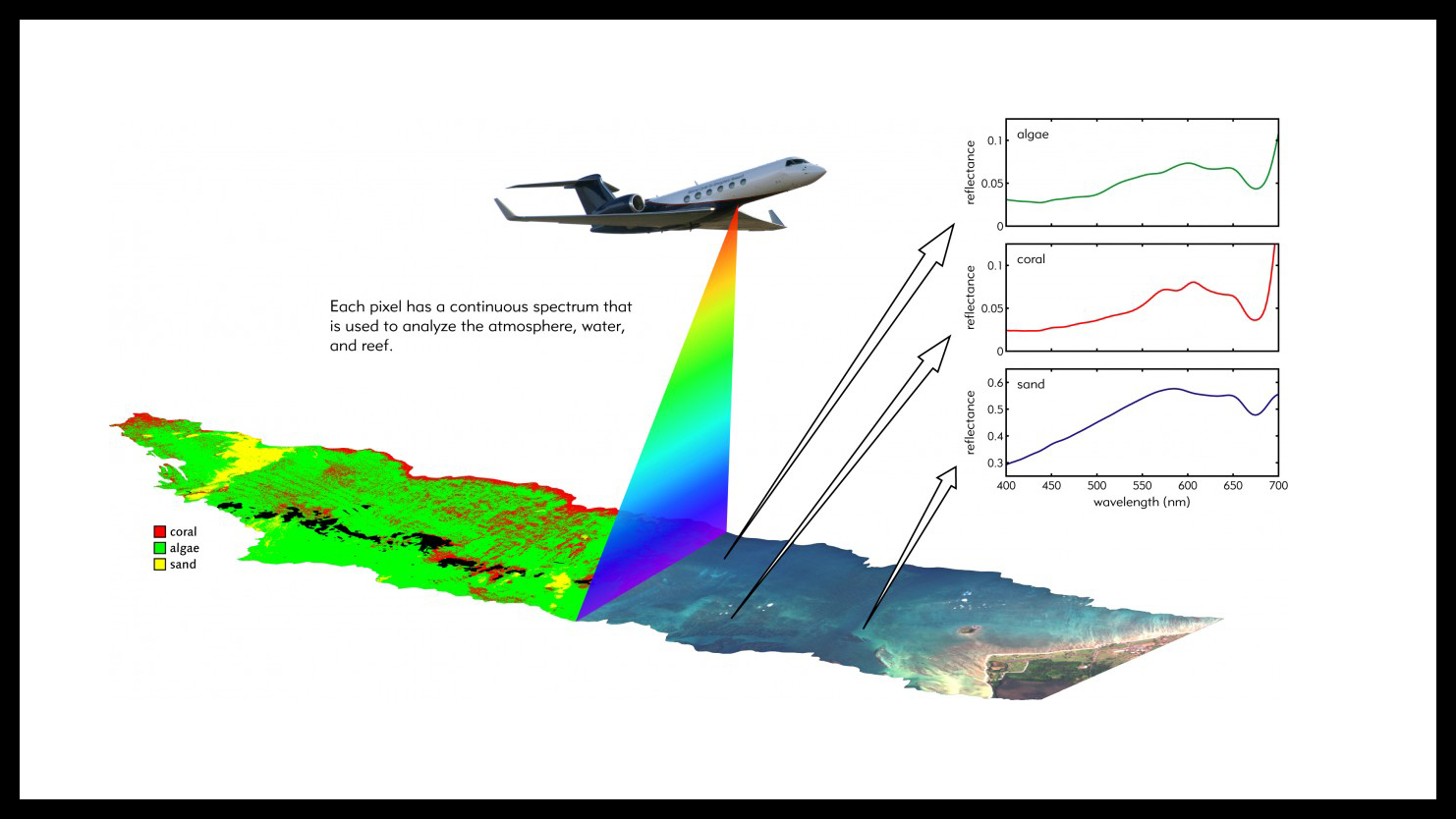
The CORAL mission records the spectra of light reflected from the ocean to study the composition and health of Earth's coral reefs. Image credit: NASA | + Expand image
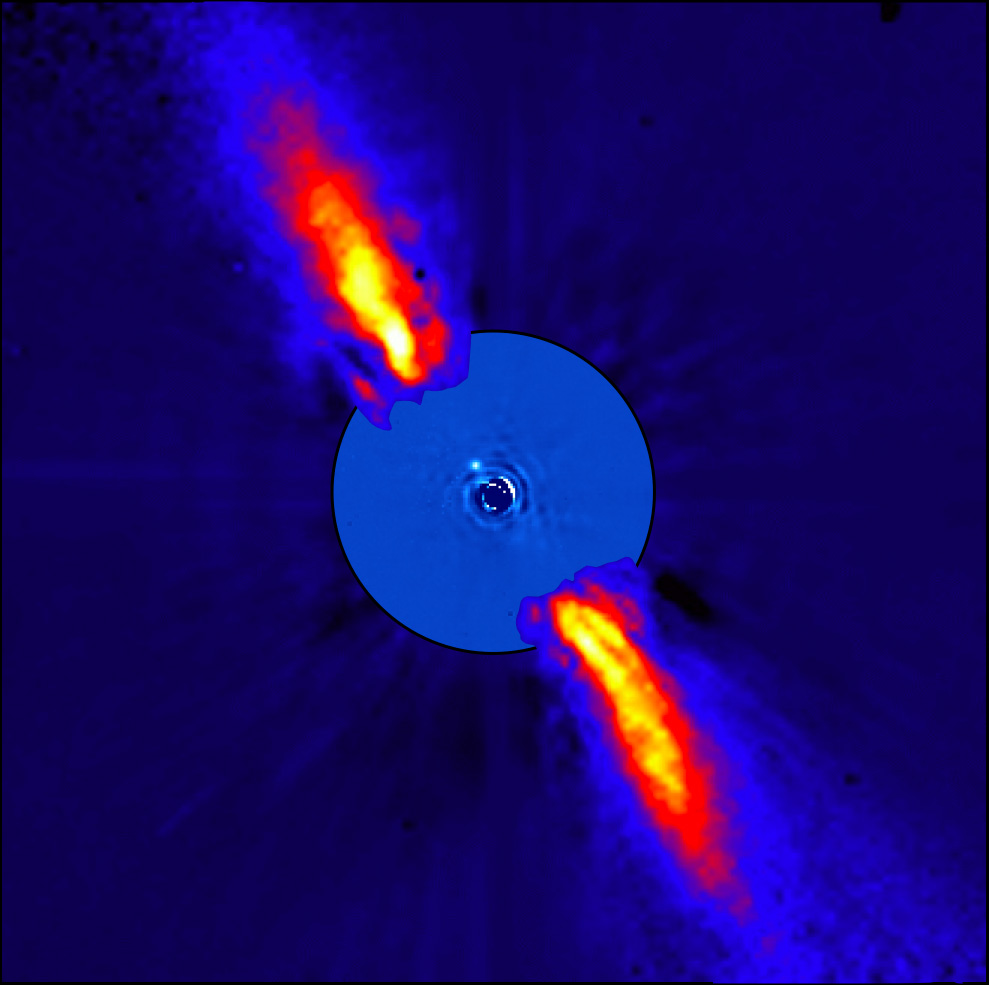
The star Beta Pictoris and its surrounding debris disk in near-infrared light. Image credit: ESO/A.-M. Lagrange et al. | › Full image and caption
Besides providing an excuse to eat all varieties of pie, Pi Day gives us a chance to appreciate some of the ways NASA uses pi to explore the solar system and beyond. You can do the math for yourself – or get students doing it – by taking part in the NASA Pi Day Challenge. Find out below how to test your pi skills with real-world problems faced by NASA space explorers, plus get lessons and resources for educators.
How It Works
The ratio of any circle's circumference to its diameter is equal to pi, which is often rounded to 3.14. But pi is what is known as an irrational number, so its decimal representation never ends, and it never repeats. Though it has been calculated to trillions of digits, we use far fewer at NASA.
Pi is useful for all sorts of things, like calculating the circumference and area of circular objects and the volume of cylinders. That's helpful information for everyone from farmers irrigating crops to tire manufacturers to soup-makers filling their cans. At NASA, we use pi to calculate the densities of planets, point space telescopes at distant stars and galaxies, steer rovers on the Red Planet, put spacecraft into orbit and so much more! With so many practical applications, it's no wonder so many people love pi!
In the U.S., 3.14 is also how we refer to March 14, which is why we celebrate the mathematical marvel that is pi on that date each year. In 2009, the U.S. House of Representatives passed a resolution officially designating March 14 as Pi Day and encouraging teachers and students to celebrate the day with activities that teach students about pi.
The NASA Pi Day Challenge
This year's NASA Pi Day Challenge poses four puzzlers that require pi to compare the sizes of Mars landing areas, calculate the length of a year for one of the most distant objects in the solar system, measure the depth of the ocean from an airplane, and determine the diameter of a distant debris disk. Learn more about the science and engineering behind the problems below or click the link to jump right into the challenge.
› Take the NASA Pi Day Challenge
› Educators, get the lesson here!
Mars Maneuver
Long before a Mars rover touches down on the Red Planet, scientists and engineers must determine where to land. Rather than choosing a specific landing spot, NASA selects an area known as a landing ellipse. A Mars rover could land anywhere within this ellipse. Choosing where the landing ellipse is located requires compromising between getting as close as possible to interesting science targets and avoiding hazards like steep slopes and large boulders, which could quickly bring a mission to its end. In the Mars Maneuver problem, students use pi to see how new technologies have reduced the size of landing ellipses from one Mars rover mission to the next.
Cold Case
In January 2019, NASA's New Horizons spacecraft sped past Arrokoth, a frigid, primitive object that orbits within the Kuiper Belt, a doughnut-shaped ring of icy bodies beyond the orbit of Neptune. Arrokoth is the most distant Kuiper Belt object to be visited by a spacecraft and only the second object in the region to have been explored up close. To get New Horizons to Arrokoth, mission navigators needed to know the orbital properties of the object, such as its speed, distance from the Sun, and the tilt and shape of its orbit. This information is also important for scientists studying the object. In the Cold Case problem, students can use pi to determine how long it takes the distant object to make one trip around the Sun.
Coral Calculus
Coral reefs provide food and shelter to many ocean species and protect coastal communities against extreme weather events. Ocean warming, invasive species, pollutants, and acidification caused by climate change can harm the tiny living coral organisms responsible for building coral reefs. To better understand the health of Earth's coral reefs, NASA's COral Reef Airborne Laboratory, or CORAL, mission maps them from the air using spectroscopy, studying how light interacts with the reefs. To make accurate maps, CORAL must be able to differentiate among coral, algae and sand on the ocean floor from an airplane. And to do that, it needs to calculate the depth of the ocean at every point it maps by measuring how much sunlight passes through the ocean and is reflected upward from the ocean floor. In Coral Calculus, students use pi to measure the water depth of an area mapped by the CORAL mission and help scientists better understand the status of Earth's coral reefs.
Planet Pinpointer
Our galaxy contains billions of stars, many of which are likely home to exoplanets – planets outside our solar system. So how do scientists decide where to look for these worlds? Using data gathered by NASA's Spitzer Space Telescope, researchers found that they're more likely to find giant exoplanets around young stars surrounded by debris disks, which are made up of material similar to what's found in the asteroid belt and Kuiper Belt in our solar system. Sure enough, after discovering a debris disk around the star Beta Pictoris, researchers later confirmed that it is home to at least two giant exoplanets. Learning more about Beta Pictoris' debris disk could give scientists insight into the formation of these giant worlds. In Planet Pinpointer, put yourself in the role of a NASA scientist to learn more about Beta Pictoris' debris disk, using pi to calculate the distance across it.
Participate
-
Pi Day Challenge Lessons
Here's everything you need to bring the NASA Pi Day Challenge into the classroom.
Grades 4-12
Time Varies
-
Slideshow: NASA Pi Day Challenge
The entire NASA Pi Day Challenge collection can be found in one, handy slideshow for students.
Grades 4-12
Time Varies
-
Pi Day: What’s Going ’Round
Tell us what you’re up to this Pi Day and share your stories and photos with NASA.
Join the conversation and share your Pi Day Challenge answers with @NASAJPL_Edu on social media using the hashtag #NASAPiDayChallenge
Blogs and Features
-
How Many Decimals of Pi Do We Really Need?
While you may have memorized more than 70,000 digits of pi, world record holders, a JPL engineer explains why you really only need a tiny fraction of that for most calculations.
-
Slideshow: 18 Ways NASA Uses Pi
Whether it's sending spacecraft to other planets, driving rovers on Mars, finding out what planets are made of or how deep alien oceans are, pi takes us far at NASA. Find out how pi helps us explore space.
Related Lessons for Educators
-
Rover Lessons
Explore a collection of standards-aligned STEM lessons all about rovers.
Grades K-12
Time Varies
-
Touchdown
Students design and build a shock-absorbing system that will protect two "astronauts" when they land.
Grades 3-8
Time 30 mins - 1 hr
-
On Target
Students modify a paper cup so it can zip down a line and drop a marble onto a target.
Grades 6-12
Time 30 mins - 1 hr
-
Solar System Scale Models
Explore a collection of standards-aligned STEM lessons all about the size and scale of our solar system.
Grades 1-12
Time Varies
-
Modeling an Asteroid
Lead a discussion about asteroids and their physical properties, then have students mold their own asteroids out of clay.
Grades 3-5
Time 30 mins - 1 hr
-
Math Rocks: A Lesson in Asteroid Dynamics
Students use math to investigate a real-life asteroid impact.
Grades 8-12
Time 30 mins - 1 hr
-
Asteroid Ace: A 'Pi in the Sky' Math Challenge
Students use pi to calculate the rotation rate of an asteroid from another solar system in this illustrated math problem.
Grades 11-12
Time < 30 mins
-
Climate Change Lessons
Explore a collection of standards-aligned STEM lessons all about Earth's changing climate.
Grades K-12
Time Varies
-
Using Light to Study Planets
Students build a spectrometer using basic materials as a model for how NASA uses spectroscopy to determine the nature of elements found on Earth and other planets.
Grades 6-11
Time < 2 hrs
-
Solar Sleuth: A 'Pi in the Sky' Math Challenge
In this illustrated math problem, students use pi and data from the Kepler space telescope to find the size of a planet outside our solar system.
Grades 6-9
Time < 30 mins
-
Exploring Exoplanets with Kepler
Students use math concepts related to transits to discover real-world data about Mercury, Venus and planets outside our solar system.
Grades 6-12
Time 30 mins - 1 hr
-
Habitable Hunt: A 'Pi in the Sky' Math Challenge
In this illustrated math problem, students use the mathematical constant pi to find the "habitable zone" around a distant star and determine which of its planets are in that zone.
Grades 11-12
Time < 30 mins
Related Activities for Students
-
Make a Moon or Mars Rover Game
Create a Moon or Mars exploration game using Scratch, a visual programming language. Think like NASA space-mission planners to design your game!
Type Project
Subject Technology
-
Make a Cardboard Rover
Build a rubber-band-powered rover that can scramble across a room.
Type Project
Subject Engineering
-
Mars in a Minute: How Do You Choose a Landing Site?
So, you want to study Mars with a lander or rover – but where exactly do you send it? Learn how scientists and engineers tackle the question of where to land on Mars in this 60-second video.
Type Video
Subject Engineering
-
Mars in a Minute: How Do You Land on Mars?
Getting a spacecraft to Mars is one thing. Getting it safely to the ground is a whole other challenge! This 60-second video from NASA's Jet Propulsion Laboratory explains three ways to land on the surface of the Red Planet.
Type Video
Subject Engineering
-
What's That Space Rock?
Find out how to tell the difference between asteroids, comets, meteors, meteorites and other bodies in our solar system.
Type Slideshow
Subject Science
-
Mars in a Minute: How Long is a Year on Mars?
How long is does it take Mars to make one trip around the Sun and why is one Earth year shorter? Find out in one minute!
Type Video
Subject Science
-
Space Place in a Snap: The Solar System's Formation
Find out how our solar system formed and how it came to be the busy place it is today.
Type Video
Subject Science
-
What Is the Kuiper Belt?
Learn about the Kuiper Belt and some of its famous members, Kuiper Belt Objects.
Type Article
Subject Science
-
Coral Bleaching Simulator
Adjust water temperature and pollution levels in this simulator to see what happens to a coral reef depending on the conditions you choose!
Type Interactive
Subject Science
-
Ocean Worlds
Where might oceans – and living things – exist beyond Earth? Scientists have their eyes on these places in our own solar system.
Type Slideshow
Subject Science
-
NASA's Earth Minute: Mission to Earth?
NASA doesn't just explore outer space! It studies Earth, too, with a fleet of spacecraft and scientists far and wide.
Type Video
Subject Science
-
NASA's Earth Minute: Earth Has a Fever
Why is Earth getting hotter and what does that mean for us?
Type Video
Subject Science
NOAA Video Series: Coral Comeback
- Article: Giant Exoplanet Hunters: Look for Debris Disks
- Video: The Evolution of a Planet-Forming Disk
- Video: Birth of "Phoenix" Planets?
Multimedia
-
Infographic: Planet Pi
This poster shows some of the ways NASA scientists and engineers use the mathematical constant pi (3.14) and includes common pi formulas.
- Posters: Exoplanet Travel Bureau
Facts and Figures
Missions and Instruments
Websites
TAGS: K-12 Education, Math, Pi Day, Pi, NASA Pi Day Challenge, Events, Space, Educators, Teachers, Parents, Students, STEM, Lessons, Problem Set, Mars 2020, Perseverance, Curiosity, Mars rovers, Mars landing, MU69, Arrokoth, New Horizons, Earth science, Climate change, CORAL, NASA Expeditions, coral reefs, oceans, Spitzer, exoplanets, Beta Pictoris, stars, universe, space telescope, Climate TM
Teachable Moments | January 23, 2020
NASA Says Goodbye to Space Telescope Mission That Revealed the Hidden Universe
In the News
On Jan. 30, 2020, the venerable Spitzer Space Telescope mission will officially come to an end as NASA makes way for a next-generation observatory. For more than 16 years, Spitzer has served as one of NASA’s four Great Observatories, surveying the sky in infrared. During its lifetime, Spitzer detected planets and signs of habitability beyond our solar system, returned stunning images of regions where stars are born, spied light from distant galaxies formed when the universe was young, and discovered a huge, previously-unseen ring around Saturn. Read on to learn more about this amazing mission and gather tools to teach your students that there truly is more than meets the eye in the infrared universe!
How It Worked
Human eyes can see only the portion of the electromagnetic spectrum known as visible light. This is because the human retina can detect only certain wavelengths of light through special photoreceptors called rods and cones. Everything we see with our eyes either emits or reflects visible light. But visible light is just a small portion of the electromagnetic spectrum. To "see" things that emit or reflect other wavelengths of light, we must rely on technology designed to sense those portions of the electromagnetic spectrum. Using this specialized technology allows us to peer into space and observe objects and processes we wouldn’t otherwise be able to see.
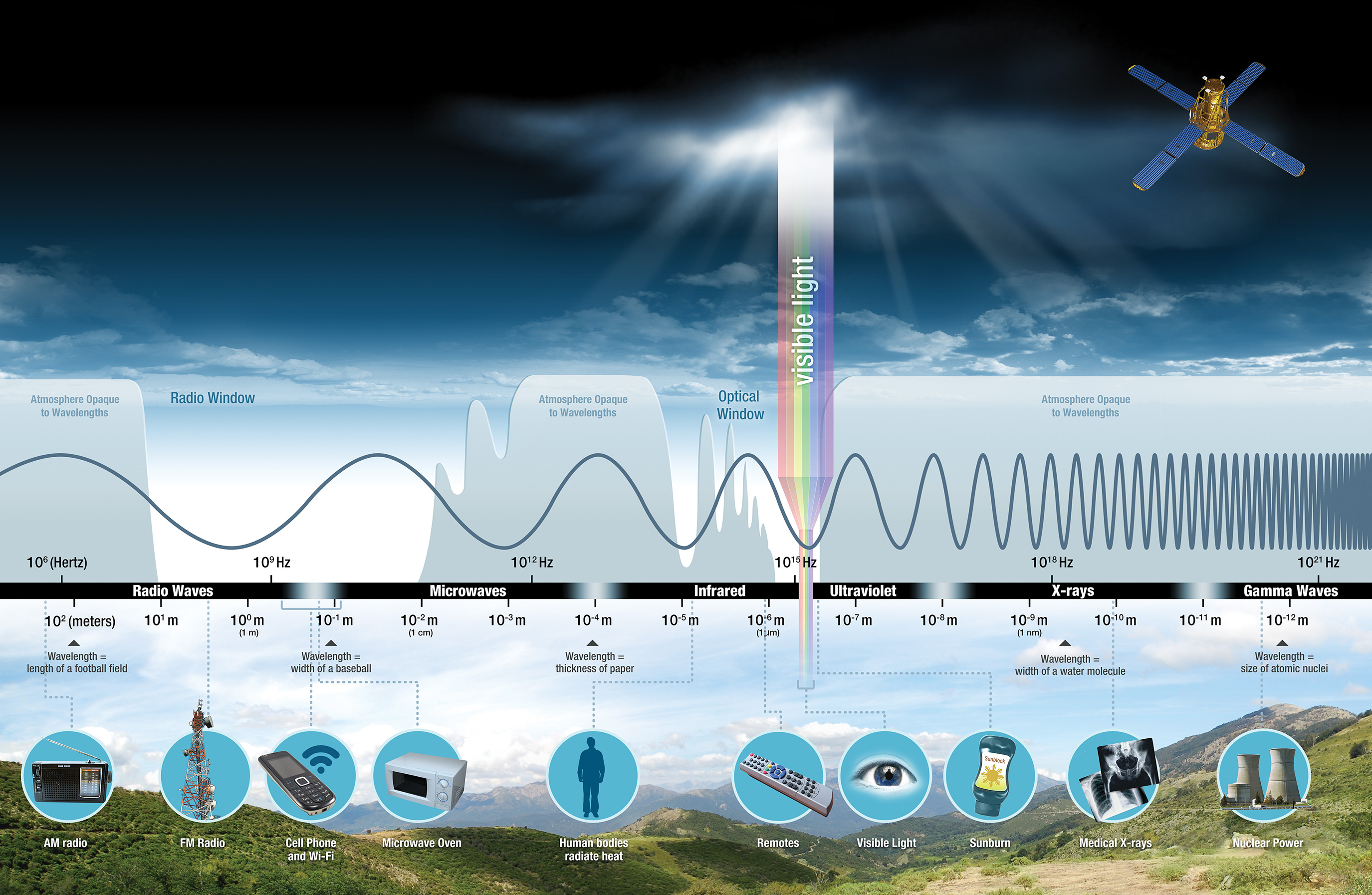
This diagram shows wavelengths of light on the electromagnetic spectrum and how they're used for various applications. Image credit: NASA | + Expand image
Infrared is one of the wavelengths of light that cannot be seen by human eyes. (It can sometimes be felt by our skin as heat if we are close enough to a strong source.) All objects that have temperature emit many wavelengths of light. The warmer they are, the more light they emit. Most things in the universe are warm enough to emit infrared radiation, and that light can be seen by an infrared-detecting telescope. Because Earth’s atmosphere absorbs most infrared radiation, infrared observations of space are best conducted from outside the planet's atmosphere.
Learn more about the infrared portion of the electromagnetic spectrum and how NASA uses it to explore space. Credit: NASA/JPL-Caltech | Watch on YouTube
So, to get a look at space objects that were otherwise hidden from view, NASA launched the Spitzer Space Telescope in 2003. Cooled by liquid helium and capable of viewing the sky in infrared, Spitzer launched into an Earth-trailing orbit around the Sun, where it became part of the agency's Great Observatory program along with the visible-light and near-infrared-detecting Hubble Space Telescope, Compton Gamma-Ray Observatory and Chandra X-ray Observatory. (Keeping the telescope cold reduces the chances of heat, or infrared light, from the spacecraft interfering with its astronomical observations.)
Over its lifetime, Spitzer has been used to detect light from objects and regions in space where the human eye and optical, or visible-light-sensing, telescopes may see nothing.
Why It's Important
NASA's Spitzer Space Telescope has returned volumes of data, yielding numerous scientific discoveries.
Vast, dense clouds of dust and gas block our view of many regions of the universe. Infrared light can penetrate these clouds, enabling Spitzer to peer into otherwise hidden regions of star formation, newly forming planetary systems and the centers of galaxies.
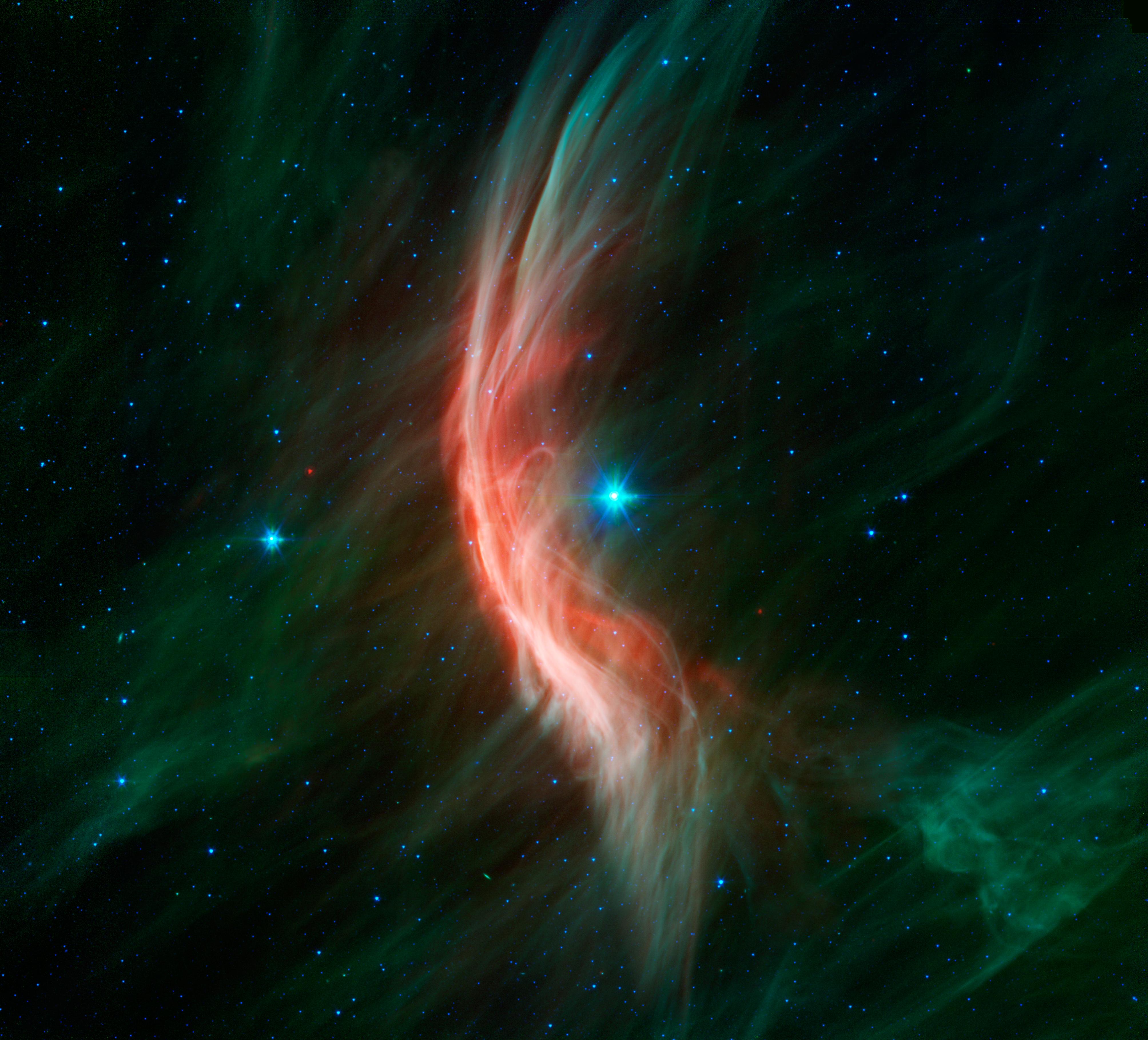
The bow shock, or shock wave, in front of the giant star Zeta Ophiuchi shown in this image from Spitzer is visible only in infrared light. The bow shock is created by winds that flow from the star, making ripples in the surrounding dust. Image credit: NASA/JPL-Caltech | › Full image and caption
Infrared astronomy also reveals information about cooler objects in space, such as smaller stars too dim to be detected by their visible light, planets beyond our solar system (called exoplanets) and giant molecular clouds where new stars are born. Additionally, many molecules in space, including organic molecules thought to be key to life's formation, have unique spectral signatures in the infrared. Spitzer has been able to detect those molecules when other instruments have not.
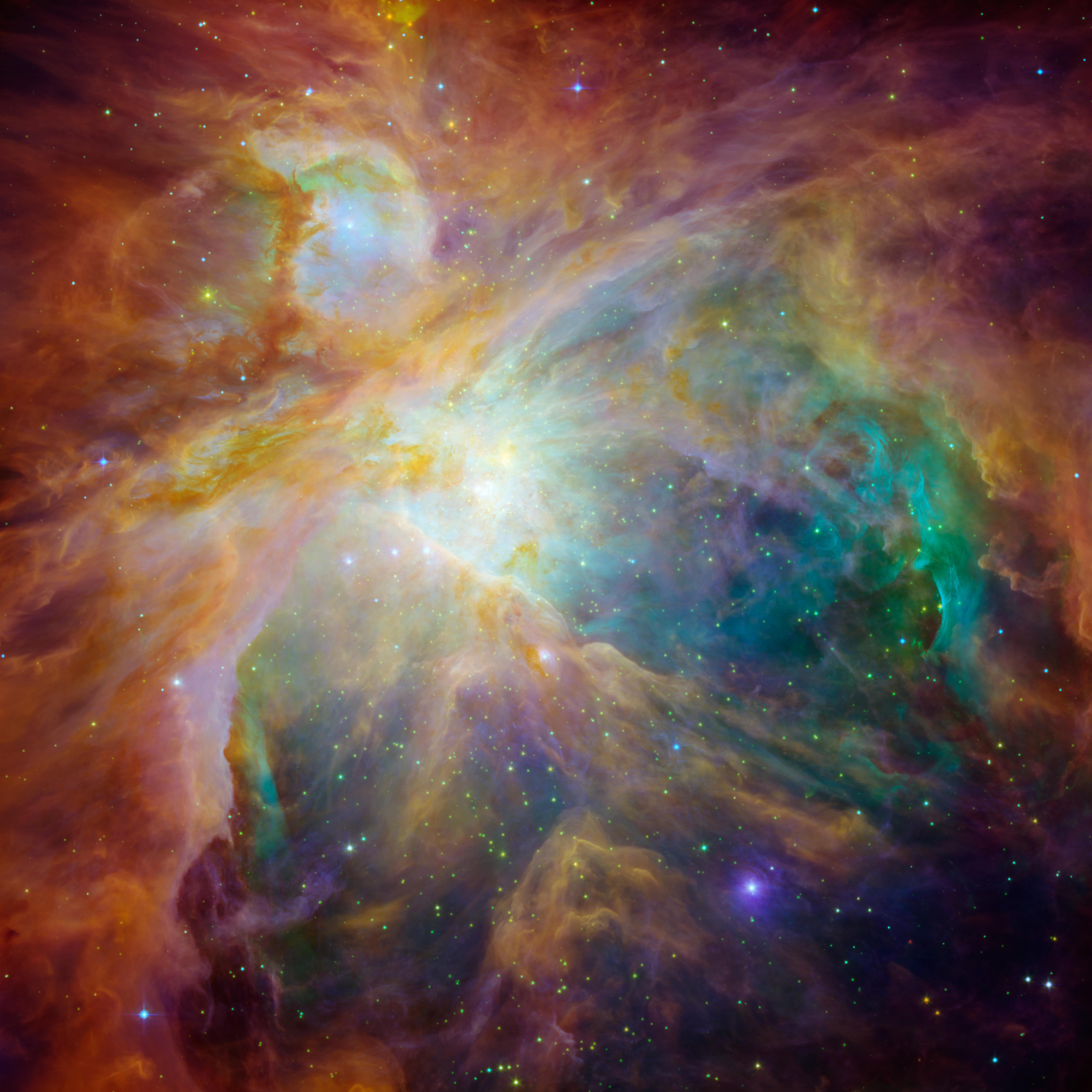
Both NASA's Spitzer and Hubble space telescopes contributed to this vibrant image of the Orion nebula. Spitzer's infrared view exposed carbon-rich molecules, shown in this image as wisps of red and orange. Image credit: NASA/JPL-Caltech/T. Megeath (University of Toledo) & M. Robberto (STScI) | › Full image and caption
Stars are born from condensing clouds of dust and gas. These newly formed stars are optically visible only once they have blown away the cocoon of dust and gas in which they were born. But Spitzer has been able to see infant stars as they form within their gas and dust clouds, helping us learn more about the life cycles of stars and the formation of solar systems.
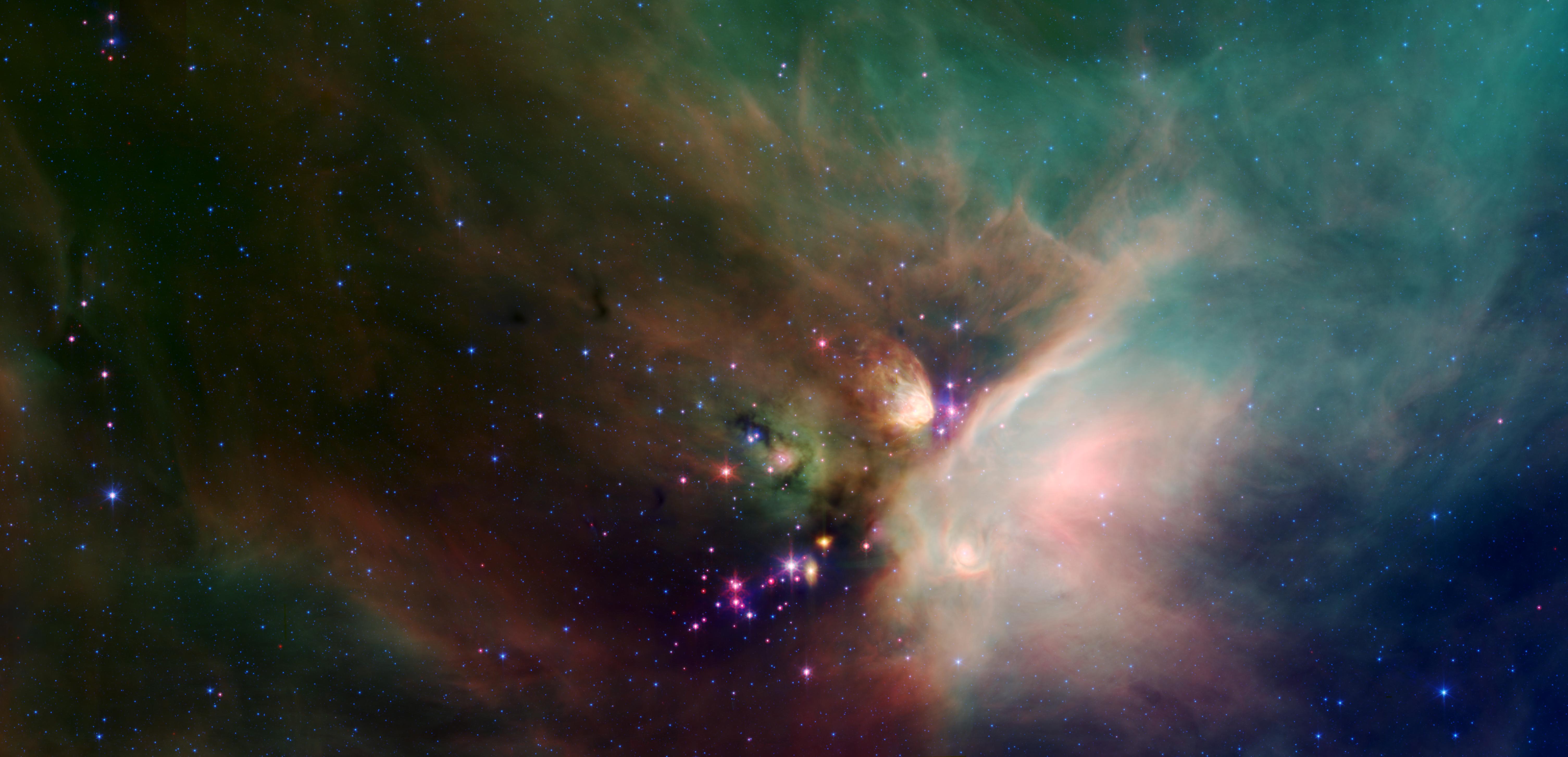
Newborn stars peek out from beneath their natal blanket of dust in this dynamic image of the Rho Ophiuchi dark cloud from Spitzer. The colors in this image reflect the relative temperatures and evolutionary states of the various stars. The youngest stars are shown as red while more evolved stars are shown as blue. Image credit: NASA/JPL-Caltech/Harvard-Smithsonian CfA | › Full image and caption
Infrared emissions from most galaxies come primarily from stars as well as interstellar gas and dust. With Spitzer, astronomers have been able to see which galaxies are furiously forming stars, locate the regions within them where stars are born and pinpoint the cause of the stellar baby boom. Spitzer has given astronomers valuable insights into the structure of our own Milky Way galaxy by revealing where all the new stars are forming.
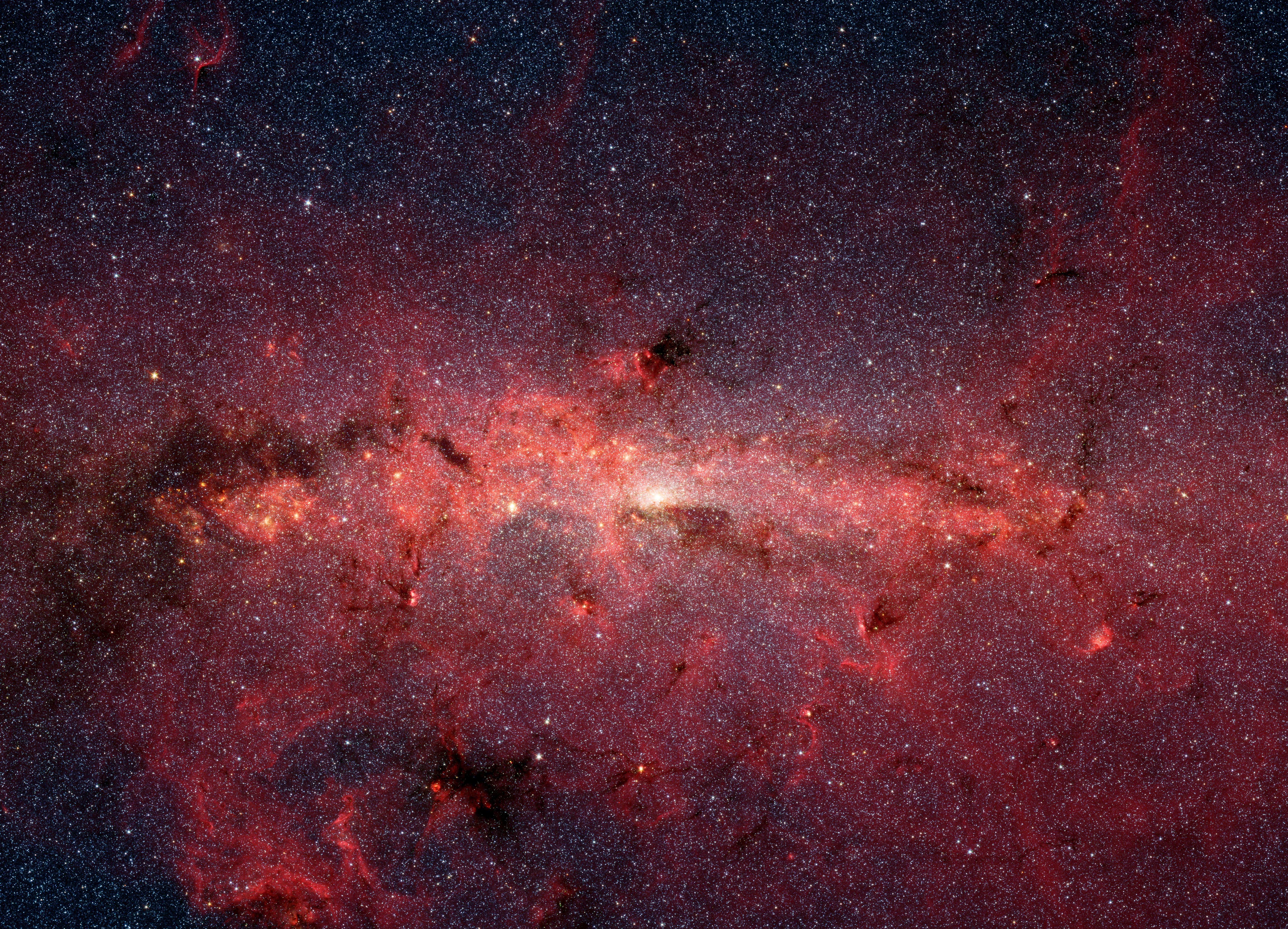
This Spitzer image, which covers a horizontal span of 890 light-years, shows hundreds of thousands of stars crowded into the swirling core of our spiral Milky Way galaxy. In visible-light pictures, this region cannot be seen at all because dust lying between Earth and the galactic center blocks our view. Image credit: NASA/JPL-Caltech | › Full image and caption
Spitzer marked a new age in the study of planets outside our solar system by being the first telescope to directly detect light emitted by these so-called exoplanets. This has made it possible for us to directly study and compare these exoplanets. Using Spitzer, astronomers have been able to measure temperatures, winds and the atmospheric composition of exoplanets – and to better understand their potential habitability. The discoveries have even inspired artists at NASA to envision what it might be like to visit these planets.
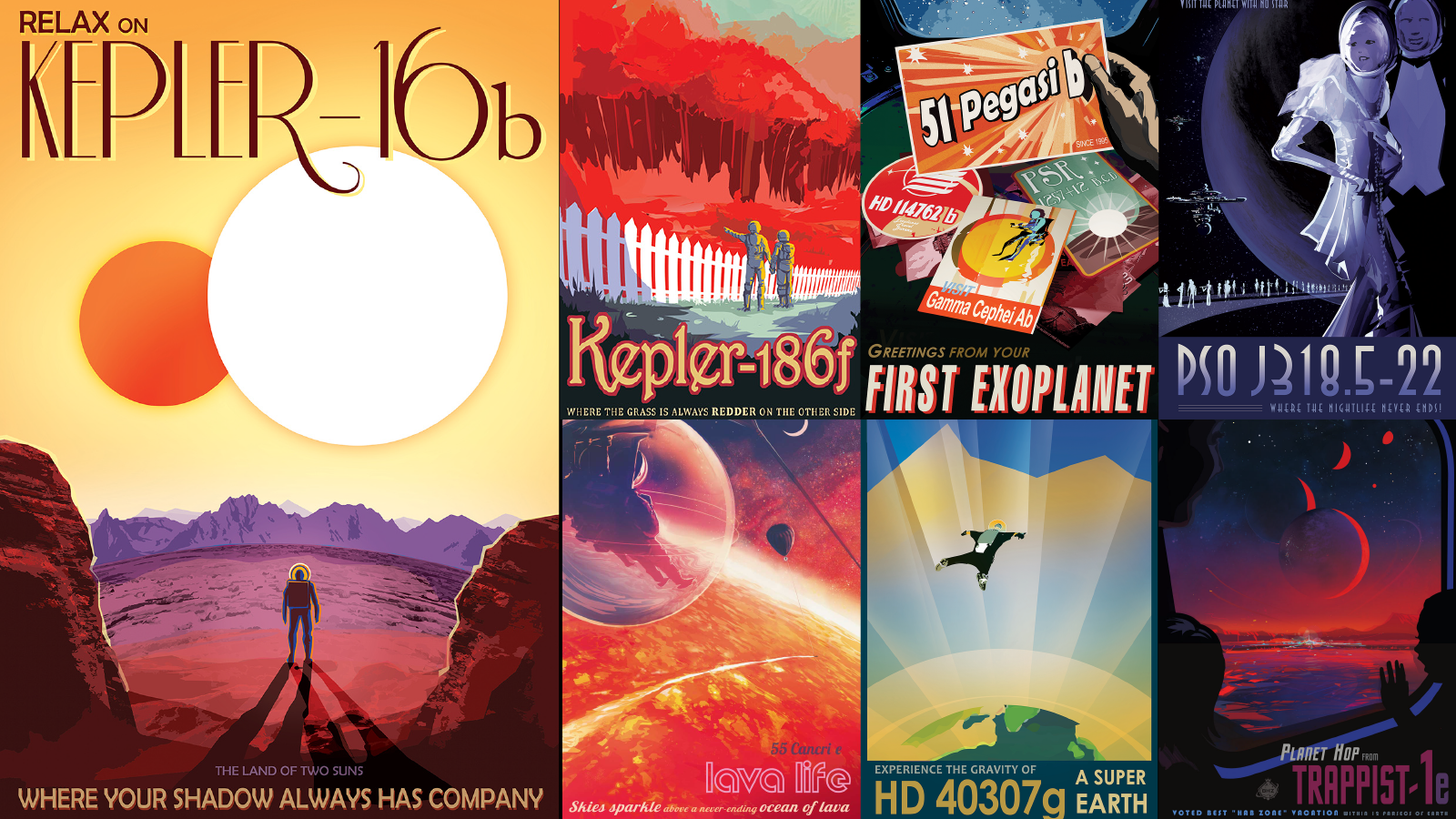
Thanks to Spitzer, scientists are learning more and more about planets beyond our solar system. These discoveries have even inspired a series of posters created by artists at NASA, who imagined what future explorers might encounter on these faraway worlds. Image credit: NASA/JPL-Caltech | › Download posters
Data collected by Spitzer will continue to be analyzed for decades to come and is sure to yield even more scientific findings. It's certainly not the end of NASA's quest to get an infrared window into our stellar surroundings. In the coming years, the agency plans to launch its James Webb Space Telescope, with a mirror more than seven times the diameter of Spitzer's, to see the universe in even more detail. And NASA's Wide Field Infrared Survey Telescope, or WFIRST, will continue infrared observations in space with improved technology. Stay tuned for even more exciting infrared imagery, discoveries and learning!
Teach It
Use these lessons, videos and online interactive features to teach students how we use various wavelengths of light, including infrared, to learn about our universe:
- Lessons: Cool Cosmos Infrared Lessons
- Website: Cool Cosmos Infrared Primer
- Materials: Infrared Posters and Printouts
Explore More
- Article: NASA Celebrates the Legacy of the Spitzer Space Telescope
- Website: Spitzer Space Telescope Mission
- Video: Spitzer Final Voyage VR 360
- Video: Science in a Minute: The Art of Spitzer Space Telescope
- Images: Spitzer Zoomable Images
- Participate: NASA/IPAC Teacher Archive Research Program
Also, check out these related resources for kids from NASA’s Space Place:
TAGS: Teachable Moments, science, astronomy, K-12 education, teachers, educators, parents, STEM, lessons, activities, Spitzer, Space Telescope, Missions, Spacecraft, Stars, Galaxies, Universe, Infrared, Wavelengths, Spectrum, Light
Teachable Moments | August 29, 2017
The Voyagers Are Still Exploring 40 Years Later
In the News
This year marks the 40th anniversary of the launch of the world’s farthest and longest-lived spacecraft, NASA’s Voyager 1 and 2. Four decades ago, they embarked on an ambitious mission to explore the giant outer planets, the two outermost of which had never been visited. And since completing their flybys of Jupiter, Saturn, Uranus and Neptune in 1989, they have been journeying toward the farthest reaches of our solar system – where no spacecraft has been before. These two intrepid spacecraft continue to return data to NASA daily, offering a window into the mysterious outer realms of our solar system and beyond.
How They Did It
The Voyager spacecraft were launched during a very short window that took advantage of a unique alignment of the four giant outer planets – one that would not occur again for another 176 years. (Try this lesson in calculating launch windows to get an idea of how it was done.) Launching at this point in time enabled the spacecraft to fly by all four planets in a single journey, returning never-before-seen, close-up images and scientific data from Jupiter, Saturn, Uranus and Neptune that greatly contributed to our current understanding of these planets and the solar system.
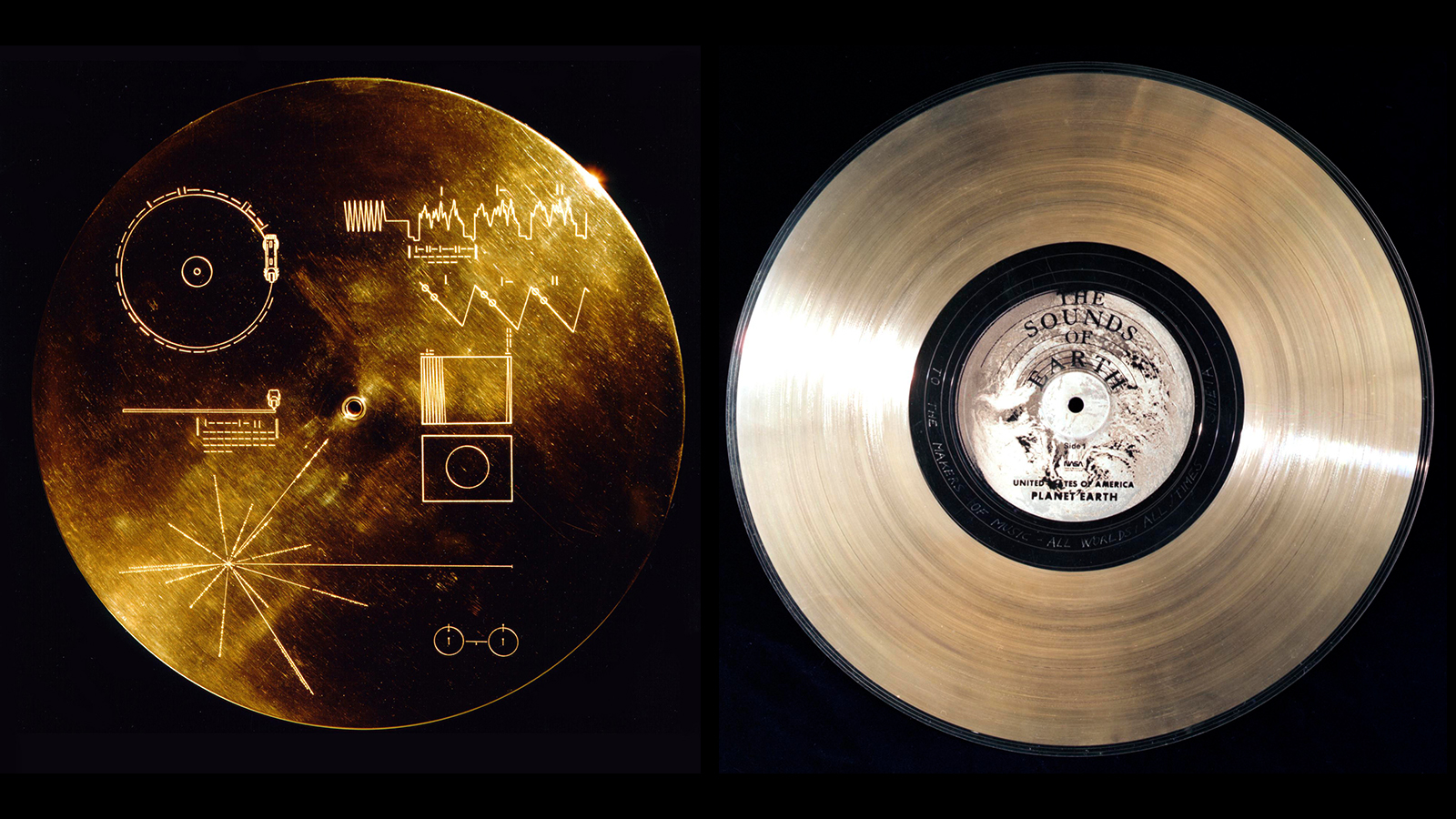
Why It’s Important
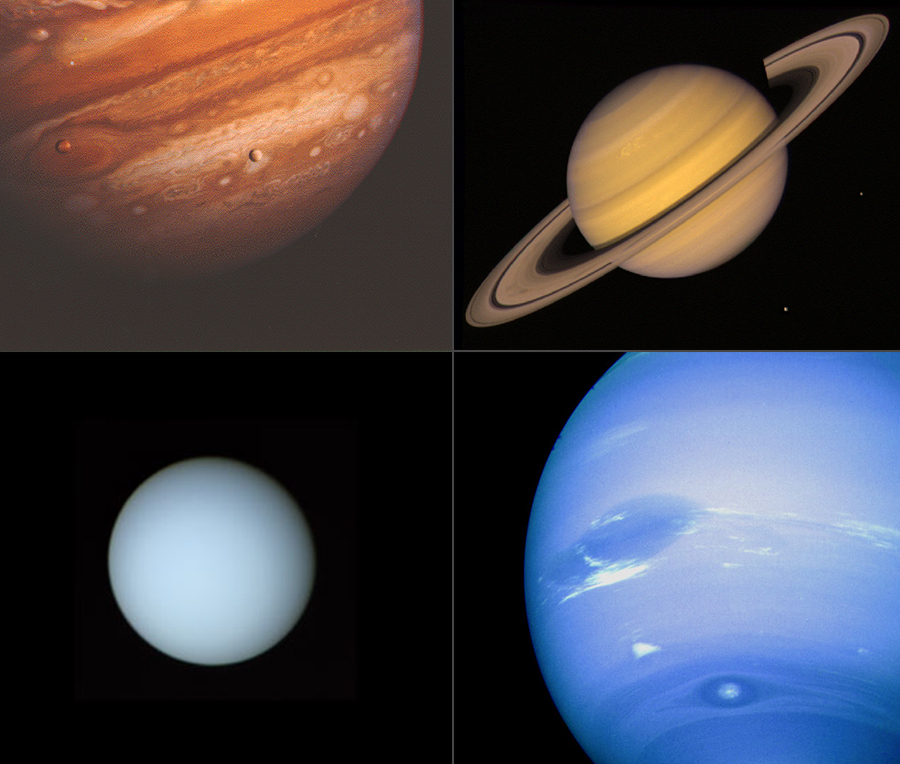
These images of Jupiter, Saturn, Uranus and Neptune (clockwise from top) were taken by Voyager 1 and 2 as the spacecraft journeyed through the solar system. See a gallery of images that Voyager took on the Voyager website. Credit: NASA/JPL-Caltech
In addition to shaping our understanding of the outer planets, the Voyager spacecraft are helping us learn more about the space beyond the planets – the outer region of our solar system. After completing their “grand tour” of the outer planets, the Voyagers continued on an extended mission to the outer solar system. They are now more than 10 billion miles from Earth, exploring the boundary region between our planetary system and what’s called interstellar space.
The beginning of interstellar space is where the constant flow of material from the Sun and its magnetic field stop influencing the surroundings. Most of the Sun’s influence is contained within the heliosphere, a bubble created by the Sun and limited by forces in interstellar space. (Note that the heliosphere doesn’t actually look like a sphere when it travels through space; it’s more of a blunt sphere with a tail.) The outer edge of the heliosphere, before interstellar space, is a boundary region called the heliopause. The heliopause is the outermost boundary of the solar wind, a stream of electrically charged atoms, composed primarily of ionized hydrogen, that stream outward from the Sun. Our planetary system lies inside the bubble of the heliosphere, bordered by the heliopause and surrounded by interstellar space.
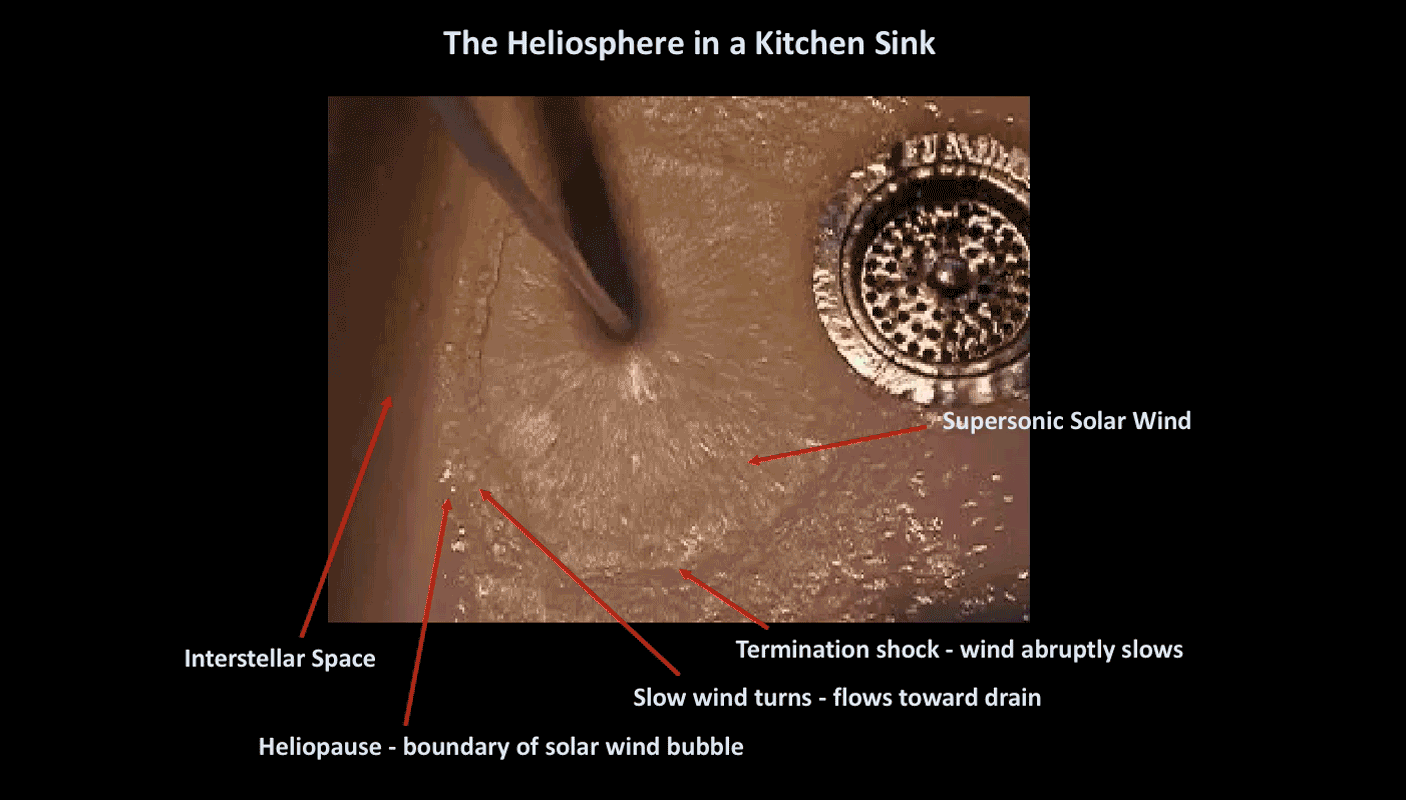
Though we’ve learned a lot about the heliopause thanks to the Voyager spacecraft, its thickness and variation are still key unanswered questions in space physics. As the Voyagers continue their journey, scientists hope to learn more about the location and properties of the heliopause.
From their unique vantage points – Voyager 1 in the northern hemisphere and Voyager 2 in the southern hemisphere – the spacecraft have already detected differences and asymmetries in the solar wind termination shock, where the wind abruptly slows as it approaches the heliopause. For example, Voyager 2 crossed the termination shock at a distance of about 83.7 AU in the southern hemisphere. (One AU, or astronomical unit, is equal to 150 kilometers (93 million miles), the distance between Earth and the Sun.) That’s about 10 AU closer to the Sun than where Voyager 1 crossed the shock in the north. As shown in this diagram, Voyager 1 traveled through the compressed “nose” of the termination shock and Voyager 2 is expected to travel through the flank of the termination shock.
With four remaining powered instruments on Voyager 1 and five remaining powered instruments on Voyager 2, the two spacecraft continue to collect science data comparing their two distinct locations at the far reaches of the solar system.

In August 2012, Voyager 1 detected a dramatic increase in galactic cosmic rays (as shown in this animated chart). The increase, which has continued to the current peak, was associated with the spacecraft's crossing into interstellar space. Credit: NASA/JPL-Caltech
Since it launched from Earth in 1977, Voyager 1 has been using an instrument to measure high-energy, dangerous particles traveling through space called galactic cosmic rays. While studying the interaction between the bubble of the heliosphere and interstellar space, Voyager 1 revealed that the heliosphere is functioning as a radiation shield, protecting our planetary system from most of these galactic cosmic rays. So in August 2012, when Voyager 1 detected a dramatic increase in the rays, which has continued to the current peak, it was associated with the spacecraft’s crossing into interstellar space.
Meanwhile, Voyager 2 – which is still in the heliosheath, the outermost layer of the heliosphere between the shock and the heliopause – is using its solar wind instrument to measure the directional change of solar wind particles there. Within the next few years, Voyager 2 is also expected to cross into interstellar space, providing us with even more detailed data about this mysterious region.
In another 10 years, we expect one or both Voyagers to cruise outward into a more pristine region of interstellar space, returning data to inform our hypotheses about the concentration of galactic particles and the characteristics of interstellar wind.
Even with 40 years of space flight behind them, the Voyagers are expected to continue returning valuable data until about 2025. Communications will be maintained until the spacecraft’s nuclear power sources can no longer supply enough electrical energy to power critical functions. Until then, there’s still much to learn about the boundary of our heliosphere and what lies beyond in the space between the stars.
Teach It
Use these standards-aligned lessons and related activities to get students doing math and science with a real-world (and space!) connection.
- Hear Here - Students use the mathematical constant pi and information about the current location of Voyager 1 to learn about the faint data-filled signal being returned to Earth.
- Solar System Bead Activity – Students calculate and construct a scale model of solar system distances using beads and string.
- Catching a Whisper from Space – Students kinesthetically model the mathematics of how NASA communicates with spacecraft.
Explore More
- Voyager Mission
- Voyager Images
- Voyager Golden Record
- The Sounds of Interstellar Space
- Voyager Senses Sun's Tsunami Wave in Interstellar Medium
- Commemorative Voyager Posters
TAGS: Voyager, Farthest, Golden Record, STEM, Teachable Moments, Science, Engineering, Solar System, Interstellar Space, Heliopause, Heliosphere, Heliosheath, Termination Shock, Stars, Heliophysics