Edu News | May 14, 2024
Our Favorite Projects for Summertime STEAM
We're launching into summer by highlighting 15 of our favorite summertime projects for students, including a Mars student challenge you can do again and again.
Just because the school year is coming to a close doesn't mean student learning has to go on vacation. In fact, with our collections of more than 100 guided out-of-school time activities and student projects that are perfect for summertime, you can find a number of ideas for keeping kids engaged while they learn about STEAM and explore NASA missions and science in the process.
Here are 15 of our favorite summer-worthy activities, plus more ways to engage students in STEAM this summer.
- Project
Make a Scale Solar System
Use beads and string, sidewalk chalk, or your own creative choice of materials to build a scale model of planet sizes or distances in the solar system.
Subject Science
Grades 2-12
Time 30-60 mins
- Project
NASA Space Voyagers: The Game
In this strategy card game, you'll build spacecraft that can explore the Moon, Mars, and other destinations throughout our solar system while withstanding challenges thrown your way.
Subject Engineering
Grades 6-12
Time 1-2 hours
- Project
Look at the Moon! Journaling Project
Draw what you see in a Moon Journal and see if you can predict the moon phase that comes next.
Subject Science
Grades K-8
Time 1-2 mins/day for 30 days
- Project
Make a Moon Phases Calendar and Calculator
Like a decoder wheel for the Moon, this calendar will show you where and when to see the Moon and every moon phase throughout the year.
Subject Science
Grades K-12
Time Less than 30 mins
- Project
Make and Code a Light-Powered Device
In this challenge, you will build and program a light-powered device that can move to collect as much light as possible while not overheating.
Subject Technology
Grades 6-12
Time 2+ hours
- Project
Make a Paper Mars Helicopter
Build a paper helicopter, then see if you can improve the design like NASA engineers did when making the first helicopter for Mars.
Subject Engineering
Grades 2-8
Time 30-60 mins
- Project
Make a Straw Rocket
Create a paper rocket that can be launched from a soda straw – then, modify the design to make the rocket fly farther!
Subject Engineering
Grades 4-8
Time Less than 30 mins
- Project
Make a Paper Glider
Turn a piece of paper into a glider inspired by a NASA design.
Subject Engineering
Grades 3-8
Time 30-60 mins
- Project
Space Origami: Make Your Own Starshade
Make your own model of this folding NASA space technology designed to help capture the first images of planets outside our solar system!
Subject Engineering
Grades 4-12
Time Less than 30 mins
- Project
Do a Mineral Mystery Experiment
Dissolve salts in water, then observe what happens when the water evaporates. Now updated with findings from Mars!
Subject Science
Grades 2-12
Time Two sessions of 30-60 mins
- Project
Make a Moon Crater
Make craters like the ones you can see on the Moon using simple baking ingredients!
Subject Science
Grades 2-8
Time 30-60 mins
- Instructor Guide
Design an Alien
Learners imagine and draw an alien that can survive with traits and environmental conditions that scientists look for in the search for life beyond Earth.
Subject Science
Grades 2-8
Time 30-60 mins
- Instructor Guide
Modeling an Asteroid
Learners imagine and draw an alien that can survive with traits and environmental conditions that scientists look for in the search for life beyond Earth.
Subject Science
Grades 3-5
Time 30-60 mins
- Instructor Guide
Model the Expanding Universe
Students learn about the role of dark energy and dark matter in the expansion of the universe, then make a model using balloons.
Subject Science
Grades 6-12
Time 30-60 mins
This last one is a great option for summer camps and other out-of-school time groups looking to fill their summer programming with STEAM. Explore seven weeks worth of lessons and activities that can be customized to your group's needs and get kids planning and designing their own mission to Mars!
Explore More
Explore the full collections of guided activities and projects at the links below:- Collection
Out-of-School Time STEAM
Explore a collection of guided STEAM activities for out-of-school time groups.
- Collection
Summertime Projects for Students
Build paper rockets and gliders, make a moon journal, write space poetry and more. These projects are the perfect way to launch into summer.
TAGS: K-12 Education, Out-of-School Time, Afterschool, Informal Education, Summer, Resources, Projects, Students, STEAM
Teachable Moments | April 22, 2024
Tracking Tiny Movements Means Big Impacts for Earth Science
Find out how the upcoming NISAR mission, an Earth satellite designed to capture detailed views of our planet's changing surface, will provide new insights into everything from natural disasters to climate change. Plus, connect it all to STEM learning.
The next addition to NASA’s fleet of Earth Science orbiters is launching in 2024 and will represent a monumental leap forward in how we monitor our changing planet. The NISAR mission is a collaboration between NASA and the Indian Space Research Organisation that’s designed to monitor and study tiny movements of Earth’s surface from events like natural disasters and climate change.
Read on to find out how NISAR is pushing the boundaries of Earth science from space. Plus, learn how you can bring science and engineering from the mission to your students.
How NISAR Works
NISAR is among the most advanced radar systems on an Earth science mission to date due to its supersized antenna reflector, use of synthetic aperture radar, and ability to observe Earth in two different radar frequencies simultaneously.
Hear mission experts describe how the NISAR satellite will track our changing Earth in fine detail. Credit: NASA/JPL-Caltech
Extending above the spacecraft like a giant catcher's mitt, NISAR’s antenna reflector is 39 feet (12 meters) wide – the largest ever launched as part of a NASA Earth-observing mission. This antenna creates an observational window, or swath, of the surface beneath the spacecraft that is 150 miles (242 kilometers) wide. The swath size is determined by the radar wavelength and antenna size, which is important because there is a direct relationship between antenna size and the resolution of images and data that can be captured by NISAR.
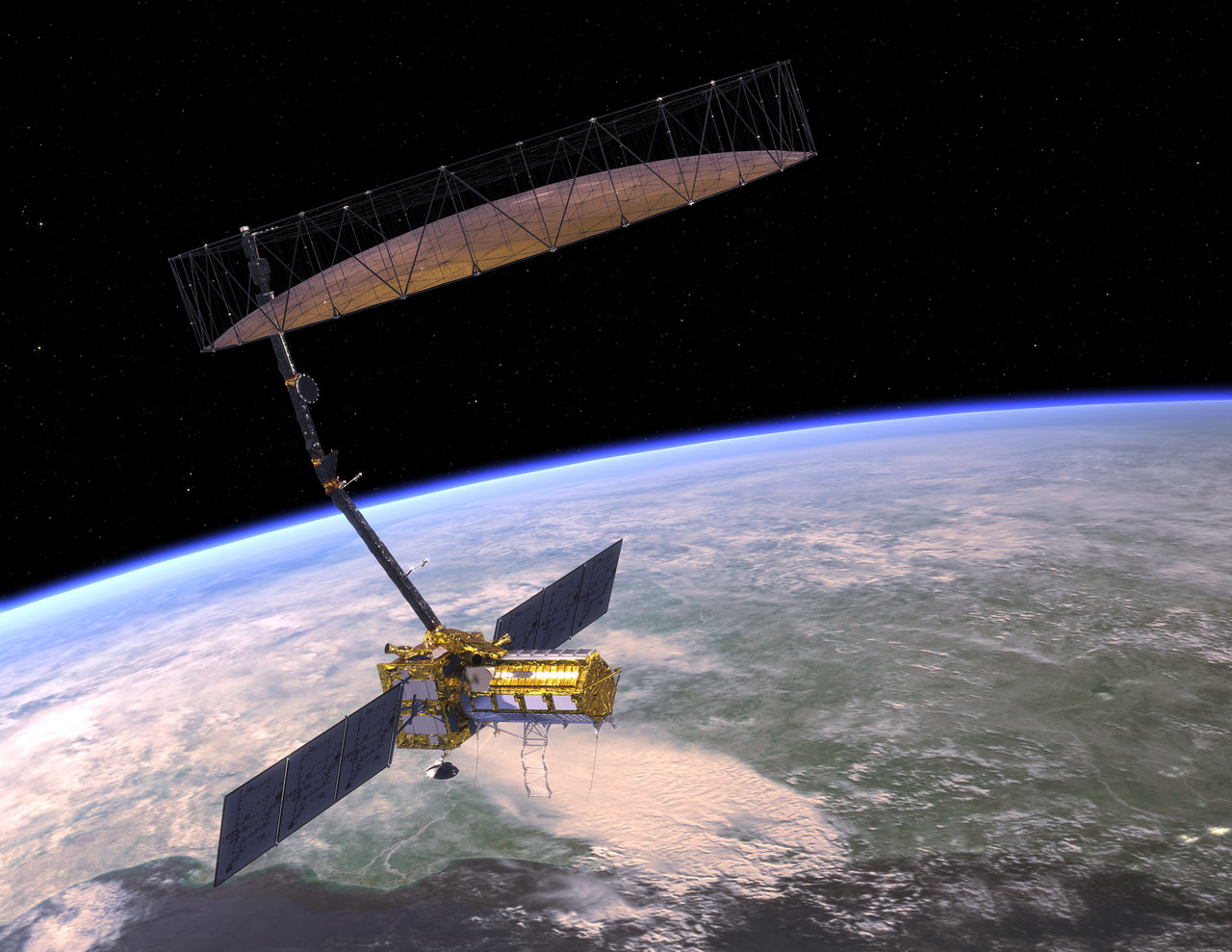
NISAR's antenna reflector extends above the spacecraft like a catcher's mitt and is engineered to help the mission get an unprecedented view of Earth's surface. Credit: NASA/JPL-Caltech | + Expand image
We typically want the best resolution possible, but we’re limited by the size of the antenna we can build and deploy in space. Conventionally, the resolution on a satellite is a function of the wavelength it uses and the size of the antenna. The larger the wavelength, the bigger the antenna needs to be to get quality images. At typical radar wavelengths, with a 12 meter diameter reflector, the best achievable resolution would be as coarse as 10s of kilometers, which is not very useful for observing features on Earth at the human scale.
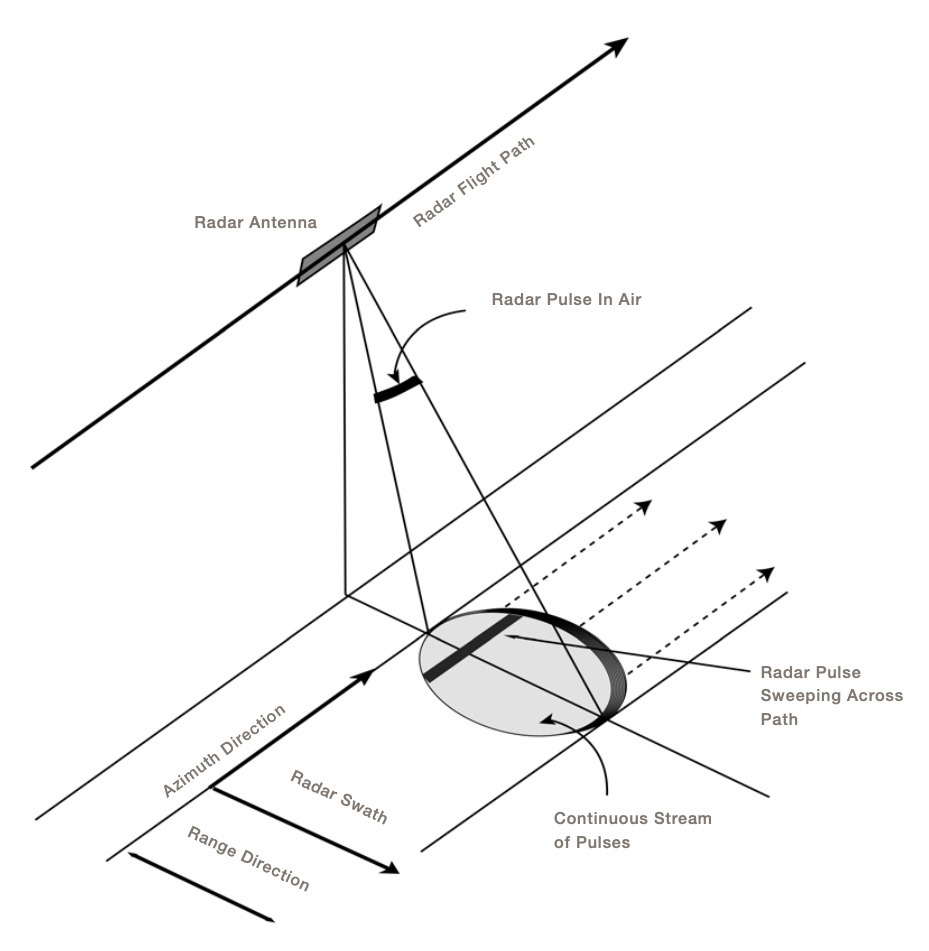
This diagram shows how synthetic aperature radar works by sending multiple radar pulses to an area on the ground from an antenna passing overhead. Credit: NASA | + Expand image
This is why NISAR utilizes an approach called synthetic aperture radar, or SAR, to synthetically magnify the resolution achievable from the antenna. With SAR, the spacecraft sends multiple signals, or pulses, to an area as it flies overhead. Each signal gets reflected back to the spacecraft, which is meticulously designed to “catch” the reflected signals thanks to its position and velocity. Each signal in the sequence is then focused into a single high-resolution image, creating an effect as if the spacecraft is using a much larger antenna.
Radar uses radio wavelengths, which are longer than those of visible light, allowing us to see through clouds and sometimes even tree coverage to the ground below, depending on the frequency of the radio waves. We’re also able to interpret a lot of information about the surface from the way the signal returns back to the orbiter. This is because NISAR will measure the amount of scatter, or dispersion, of the signal as compared to when it was originally transmitted.
For example, a rigid, sharp angled building will bounce the signal back to the receiver differently than a leafy tree. Different radio frequencies are better used for different surfaces because they are influenced by the type of surface being analyzed. To this end, NISAR is the first mission to use two different radar frequencies simultaneously. The L-Band can be used to monitor heavier vegetation and landscapes while the S-Band is better tuned for lighter vegetation and crop growth. The two wavelengths in general extend the range of sensitivity of the measurement to smaller and larger changes.
This combination of tools and features will allow NISAR to construct global maps of changes in the position of any given pixel at a scale of just centimeters as well as subtle changes in reflectivity due to land cover changes on all land and ice surfaces twice every 12 days. The resolution combined with repetition will allow scientists to monitor the changes taking place on our planet in a matter of days more comprehensively than ever before.
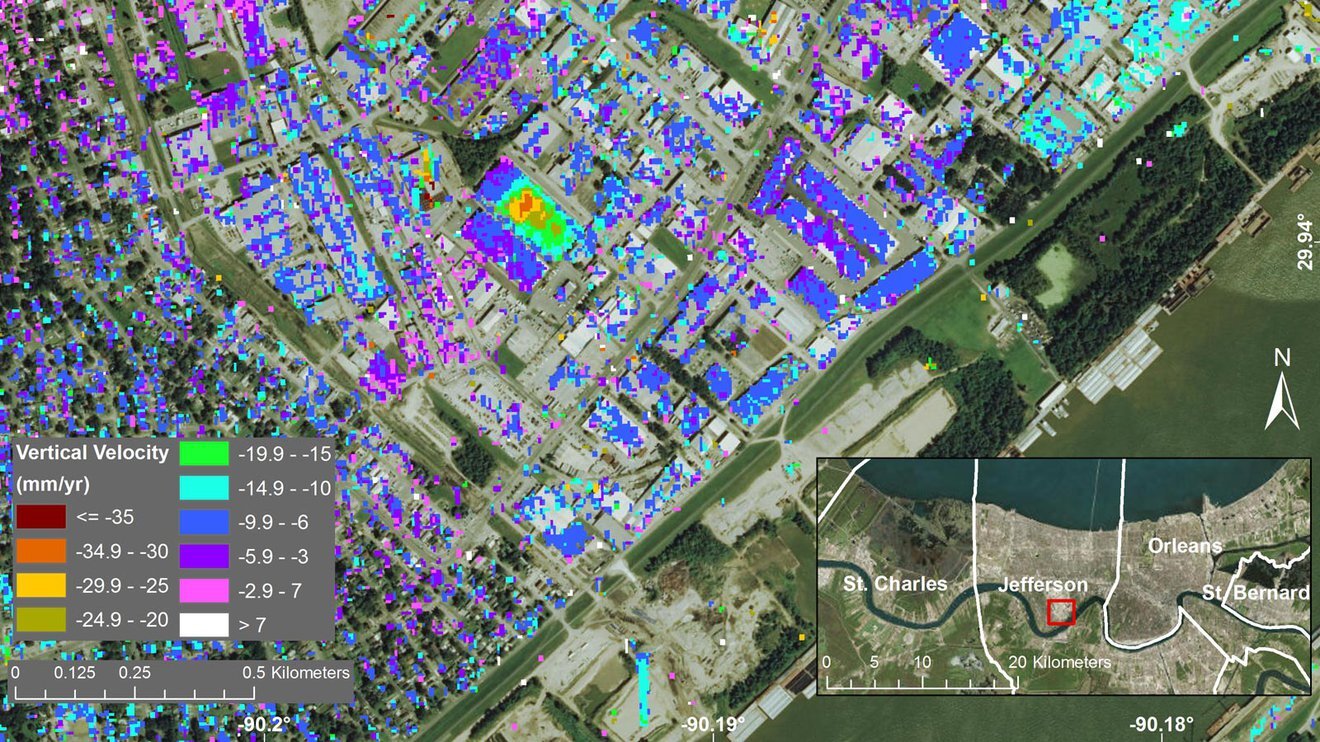
This satellite image of New Orleans is overlaid with synthetic aperature radar data from the UAVSAR instrument to show the rate at which the land was sinking in a section of New Orleans from June 2009 to July 2012. Credit: NASA/JPL-Caltech, Esri | › Learn more
What the NISAR Mission Will Show Us
Because of the massive amount of data produced by NISAR, we’ll be able to closely monitor the impacts of environmental events including earthquakes, landslides, and ice-sheet collapses. Data from NISAR could even be used to assess the risk of natural hazards.
Scientists can use NISAR to monitor tiny movements in Earth’s surface in areas prone to volcanic eruptions or landslides. These measurements are constructed using what’s called an interferogram, which looks at how the maps generated for each pass of the spacecraft have changed over time. For example, we could see immediate changes to the topography after an earthquake with an interferogram made from images NISAR collected shortly before and soon after the event.
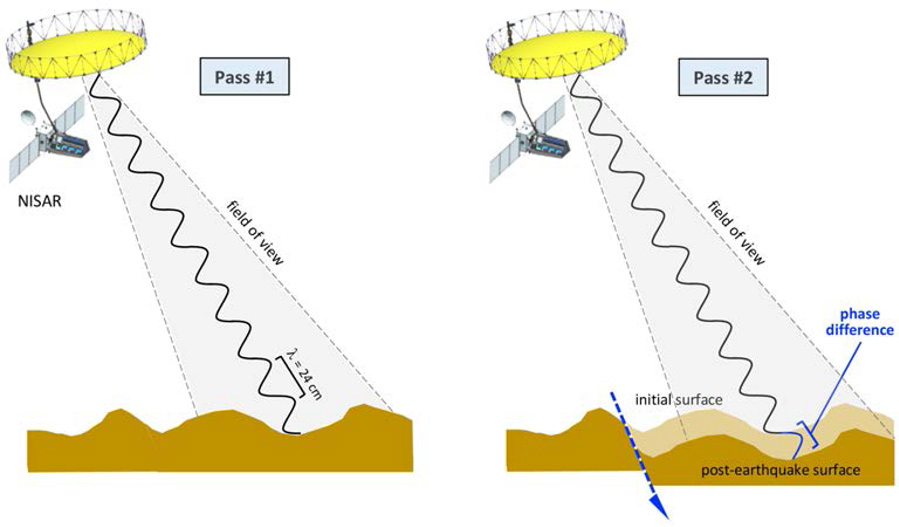
Using interferometry, as shown in this diagram, NISAR can capture changes or deformation in land surfaces, such as after an earthquake. | + Expand image
By tracking and recording these events and other movements on the surface leading up to natural disasters, it may be possible to identify warning signs that can improve detection and disaster response.
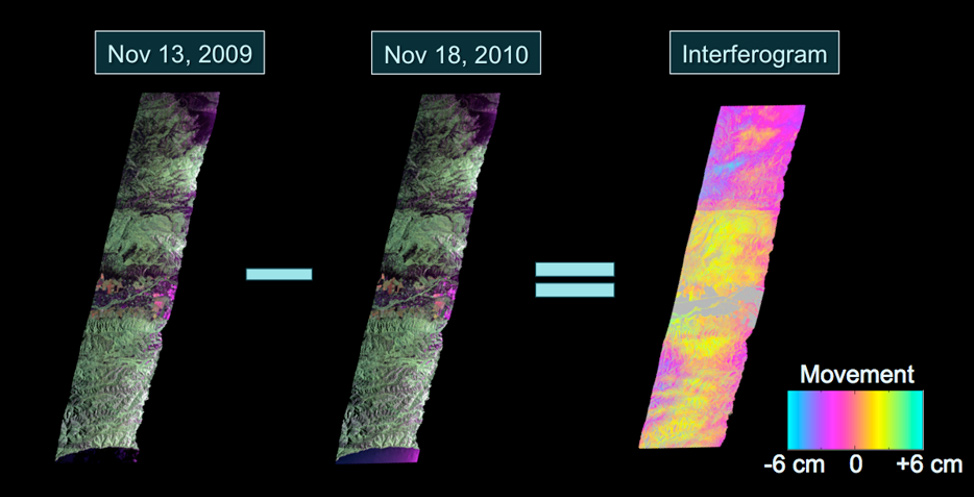
The first two images in this series were captured by the UAVSAR instrument during two separate passes over California's San Andreas Fault about a year apart. The two images were then combined to create the third image, which an interferogram that shows how the surface changed between the two passes of the instrument. Credit: NASA/JPL-Caltech | + Expand image
And NISAR isn’t just limited to studying the solid Earth. As missions prior have done, it will also be able to generate maps of polar ice sheets over time and detect changes in permafrost based on the regional movement of the soil below. These measurements will give climate scientists a clear picture of how much the ice is moving and deforming due to climate change and where it is thawing as the ground warms.
Additionally, NISAR can track land usage, deforestation, sea levels, and crustal deformation, informing scientists about the impacts of environmental and climate change on Earth.
Follow Along With NISAR
NISAR is scheduled to launch in 2024 from the Satish Dhawan Space Centre in Sriharikota, India, and will enter a polar orbit 460 miles (747 kilometers) above Earth. For the first 90 days after launch, the spacecraft will undergo checks and commissioning before beginning scientific observations for a primary mission designed to last three years.
Science from the mission will be downlinked to both NASA and ISRO ground stations below with data and the tools to process it freely available for download and use to all professional and citizen scientists.
Visit NASA’s NISAR mission page for the latest updates about the mission.
Teach Earth Science With NISAR
With the launch of NISAR, we will be better able to monitor and mitigate natural disasters and understand the effects of climate change. Bring the fleet of NASA Earth Science missions to your classroom with the following lessons and activities:
Lessons
- Math Problem
Orbit Observation: A ‘Pi in the Sky’ Math Challenge
In this illustrated math problem, students use the mathematical constant pi to figure out how much data the NISAR spacecraft collects every day.
Subject Math
Grades 7-12
Time Less than 30 mins
- Lesson
Modeling Crustal Folds
Students use playdough to model how Earth’s crust is bent and folded by tectonic plates over geologic time.
Subject Science
Grades 6-12
Time 30-60 mins
- Lesson
Making Topographic Maps
Students draw and interpret topographic maps while learning about technology used to map Earth's surface, the seafloor, and other worlds.
Subject Science
Grades 6-12
Time 1-2 hrs
- Lesson
Using Light to Study Planets
Students build a spectrometer using basic materials as a model for how NASA uses spectroscopy to determine the nature of elements found on Earth and other planets.
Subject Science
Grades 6-11
Time 2+ hrs
- Lesson
Modeling the Water Budget
Students use a spreadsheet model to understand droughts and the movement of water in the water cycle.
Subject Science
Grades 5-8
Time 30-60 mins
- Lesson
Fired Up Over Math: Studying Wildfires from Space
Students learn how scientists assess wildfires using remote sensing and solve related math problems, appropriate for various grade levels.
Subject Math
Grades 3-12
Time 30-60 mins
- Lesson
Earth Science Data Visualizations – How to Read a Heat Map
Students learn to read, interpret and compare “heat map” representations of Earth science data.
Subject Science
Grades 4-12
Time 30-60 mins
- Lesson
Lessons in Sea-Level Rise
What is sea-level rise and how does it affect us?
Subject Science
Grades 5-12
Time 30-60 mins
- Collection
Earth Science Lesson Collection
Discover a collection of standards-aligned STEM lessons all about Earth and climate change.
- Collection
Climate Change Lesson Collection
Explore a collection of standards-aligned STEM lessons for students that get them investigating climate change along with NASA.
Student Projects and Activities
- Collection
Exploring Earth Activities Collection
Try these science and engineering projects, watch videos, and explore images all about the planet that we call home.
- Collection
Climate Change Activities Collection
Learn about climate change and its impacts with these projects, videos, and slideshows for students.
Articles
- Collection
Teachable Moments in Climate Change
Explore this collection of Teachable Moments articles to get a primer on the latest NASA Earth science missions, plus find related education resources you can deploy right away!
- Collection
Earth Minute Video Series
This series of animated white-board videos for students of all ages explains key concepts about Earth science, missions, and climate change.
- Expert Talk
Monitoring Earth from Space
In this educational talk, NASA experts discuss how we build spacecraft to study climate, then answer audience questions.
- Interactive: NASA Eyes on Earth
- Images: NASA Earth Observatory
- Gallery: Images of Climate Change
- Infographic: Sea Level Rise
Videos
Explore More
Websites
Facts & Figures
Multimedia
TAGS: K-12 Education, Resources, Earth Science, Climate Change, NISAR
Teachable Moments | March 18, 2024
The Science of Solar Eclipses and How to Watch With NASA
Get ready for the April 8 total solar eclipse. Learn about the science behind solar eclipses, how to watch safely, and how to engage students in NASA science.
On April 8, 2024, a total solar eclipse will be visible across much of the central and northeastern United States, as well as parts of Mexico and Canada.
Whether you are traveling to the path of the total eclipse or will be able to step outside and watch the eclipse where you live, here's everything you need to know, including what to expect, how to watch safely, and how to engage in scientific observations and discovery with NASA.
What Are Solar Eclipses?
Solar eclipses occur when the Sun, the Moon, and Earth align. For this alignment to happen, two things need to be true. First, the Moon needs to be in the new moon phase, which is when the Moon’s orbit brings it between Earth and the Sun. Second, eclipses can only happen during eclipse seasons, which last about 34 days and occur just shy of every six months. An eclipse season is the time period when the Sun, the Moon, and Earth can line up on the same plane as Earth's orbit during a new or full moon. If a new moon happens during an eclipse season, the shadow cast by the Moon will land on Earth, resulting in a solar eclipse. Most of the time, because the Moon’s orbit is slightly tilted, the Moon’s shadow falls above or below Earth, and we don't get a solar eclipse.
Not all solar eclipses look the same. The distance between the Sun, the Moon, and Earth plays an important role in what we see during a solar eclipse. Even though the Moon is much smaller than the Sun (about 400 times smaller in diameter), the Sun and Moon look about the same size from Earth. This is because the Sun is about 400 times farther away than the Moon. But as the Moon travels its elliptical orbit around Earth, its size appears slightly larger when it is closer to Earth and slightly smaller when it is farther from Earth. This contributes to the different kinds of solar eclipses you might have heard about. For example:
- During a total solar eclipse, the Moon is closer to Earth in its orbit and appears larger, completely blocking the Sun's disk. This allows viewers in the path of totality to see the Sun’s corona, which is usually obscured by the bright light of the Sun’s surface.
- An annular solar eclipse occurs when the Sun, Moon, and Earth are properly aligned, but the Moon is farther away in its orbit, so it does not completely cover the Sun's disk from our perspective. Annular eclipses are notable for the "ring of fire," a thin ring of the Sun’s disk that's still visible around the Moon during annularity. The name annular eclipse comes from the world of mathematics, where a ring shape is known as an annulus.
- Partial eclipses can happen for two reasons. First, viewers outside the path of totality during a total solar eclipse – or the path of annularity during an annular eclipse – will see only part of the Sun’s surface covered by the Moon. The other time a partial eclipse can occur is when the Moon is nearly above or below Earth in its orbit so only part of the Moon’s shadow falls on Earth. In this case, only part of the Sun’s surface will appear covered by the Moon.
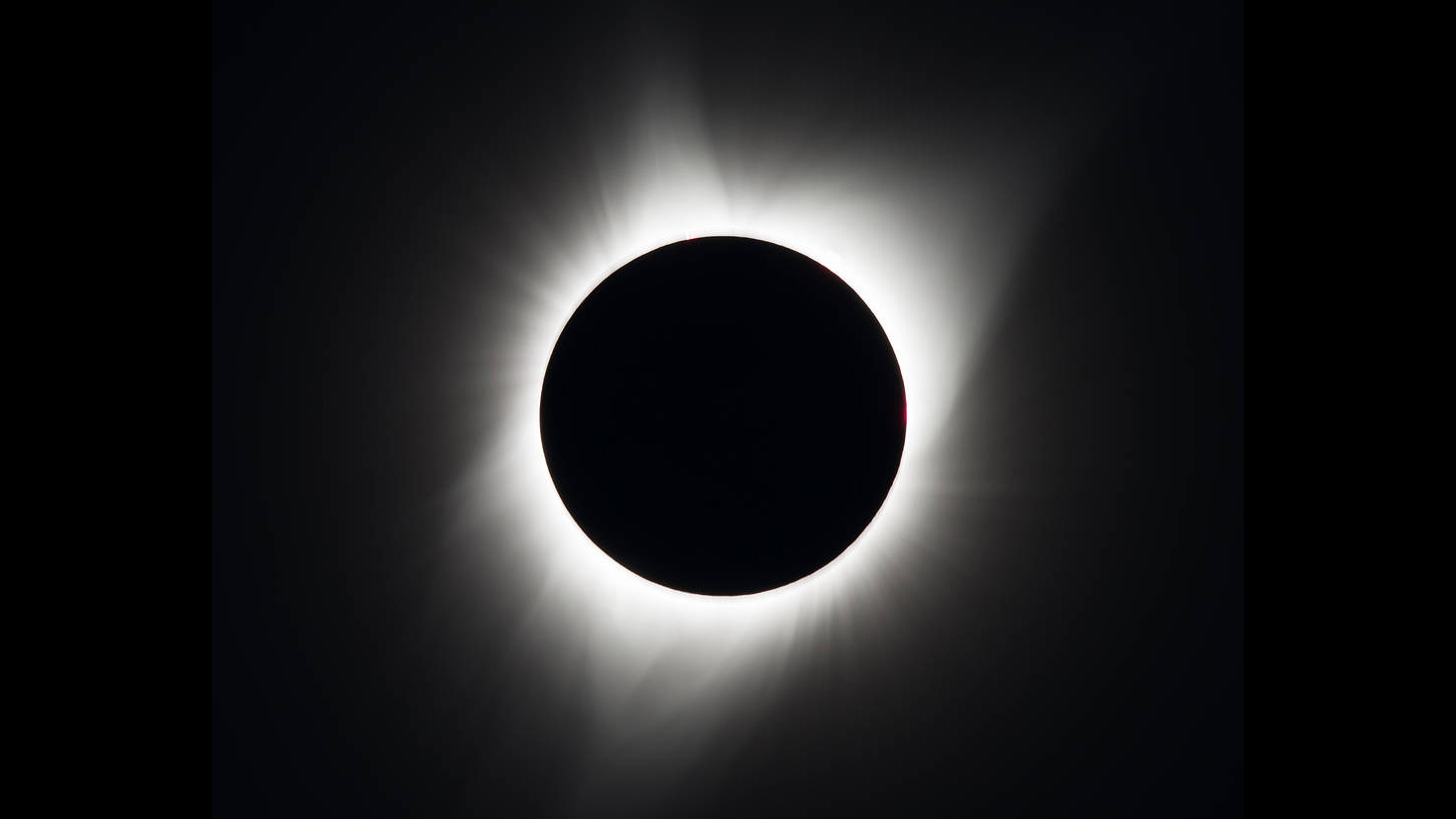
This image of a total solar eclipse was captured on Aug. 21, 2017 from Madras, Oregon. Image credit: NASA/Aubrey Gemignani | › Full image and caption
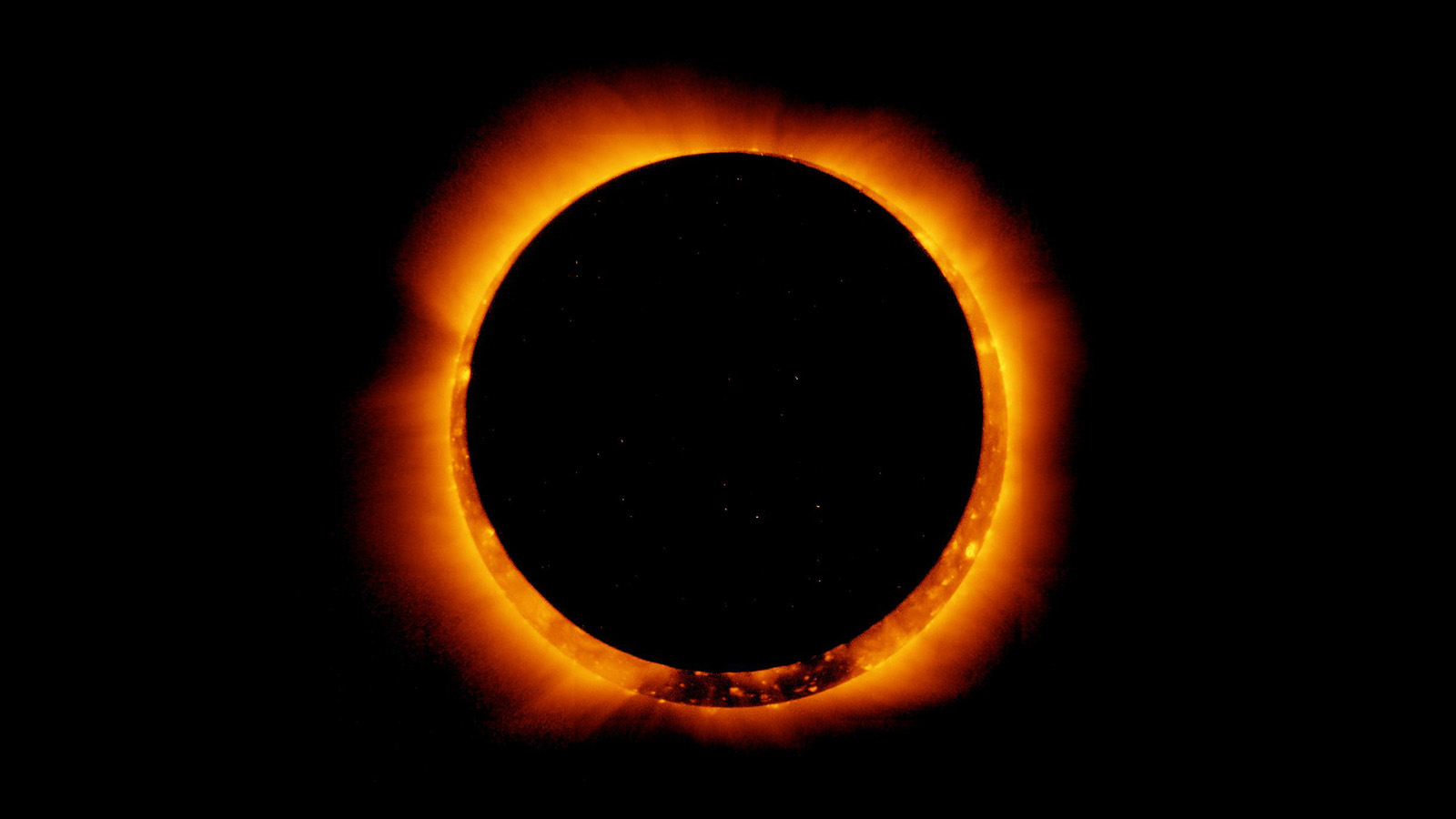
On Jan. 4, 2017, the Hinode satellite captured these breathtaking images of an annular solar eclipse. Image credit: Hinode/XRT | › Full image and caption
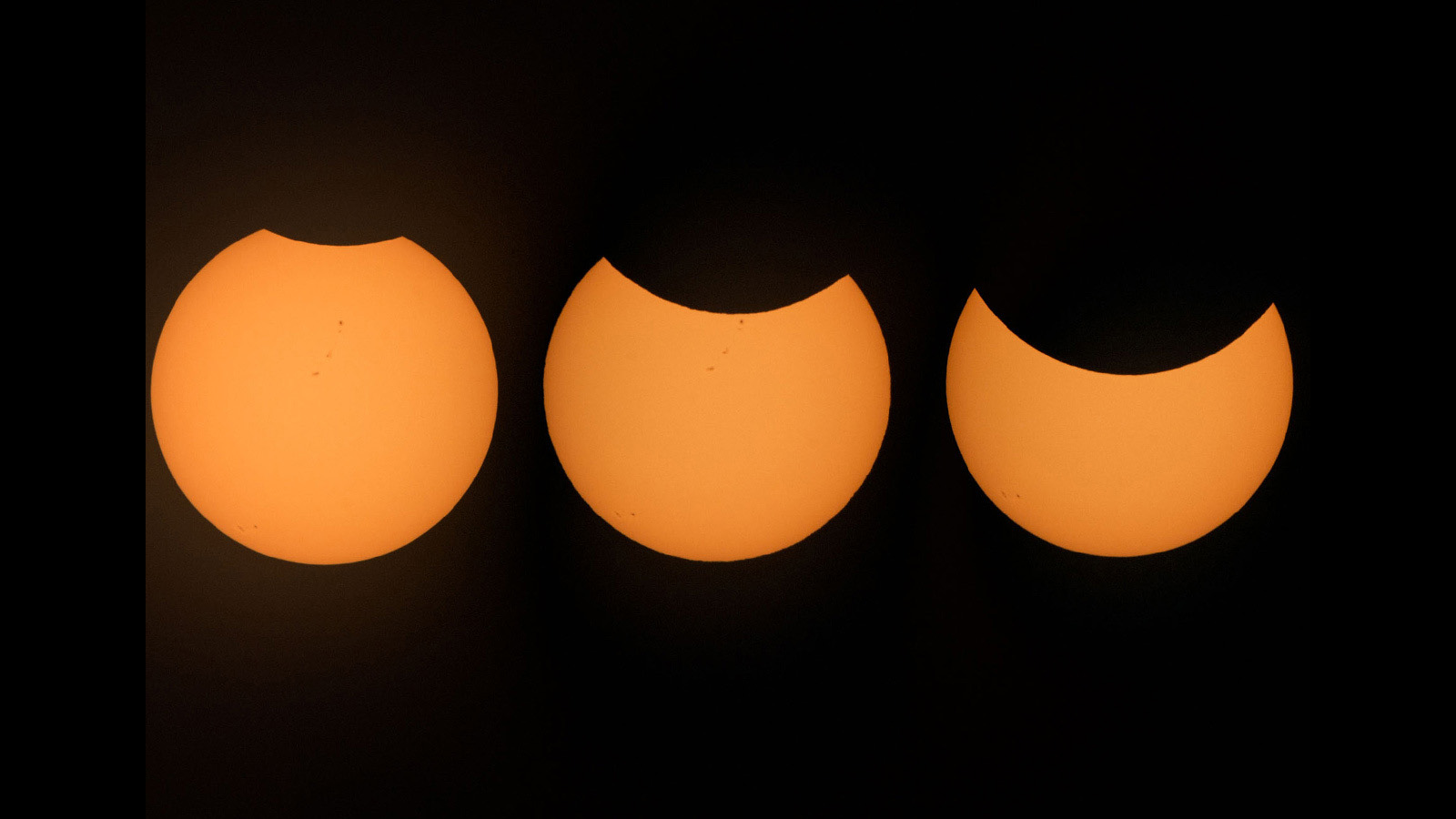
The Sun appears partially eclipsed in this series of photos taken from NASA’s Johnson Space Center in Houston on Aug. 21, 2017. Image credit: NASA/Noah Moran | › Full image and caption
How to Watch the Upcoming Solar Eclipse
First, an important safety note: Do not look directly at the Sun or view any part of the partial solar eclipse without certified eclipse glasses or a solar filter. Read more below about when you can safely view the total solar eclipse without eclipse glasses or a solar filter. Visit the NASA Eclipse website for more information on safe eclipse viewing.
When following proper safety guidelines, witnessing an eclipse is an unparalleled experience. Many “eclipse chasers” have been known to travel the world to see solar eclipses. Here's what to expect on April 8, 2024:
The start time and visibility of the eclipse will depend on your location. You can use the interactive map below to find detailed eclipse information, including timing and coverage, by entering in your location. A list of some of the cities and start times along the path of totality is available on the NASA Science website.
Explore when and where to view the eclipse with this interactive map from NASA. Enter your zip code to see what will be visible in your viewing location and when to watch. Credit: NASA's Scientific Visualization Studio
The eclipse begins when the edge of the Moon first crosses in front of the disk of the Sun. This is called a partial eclipse and might look as if a bite has been taken out of the Sun.
It is important to keep your eclipse glasses on during all parts of the partial solar eclipse. The visible part of the Sun is tens of thousands of times brighter than what you see during totality. You can also use a pinhole camera to view the eclipse.
An approximately 115-mile-wide strip known as the path of totality is where the shadow of the Moon, or umbra, will fall on Earth. Inside this path, totality will be visible starting about 65 to 75 minutes after the eclipse begins.
If you are in the path of totality, it is safe to take off your eclipse glasses and look at the total eclipse only during totality. Be sure to put your glasses back on before the total phase ends and the surface of the Sun becomes visible again. Your viewing location during the eclipse will determine how long you can see the eclipse in totality. In the U.S., viewers can expect to see 3.5 to 5.5 minutes of totality.
After totality ends, a partial eclipse will continue for 60 to 80 minutes, ending when the edge of the Moon moves off of the disk of the Sun.
For more information about the start of the partial eclipse, the start and duration of totality, and the percentage of the Sun eclipsed outside the path of totality, find your location on this eclipse map.
On April 8, NASA Television will host a live broadcast featuring expert commentary and views from telescopes along the path of totality. Tune into the broadcast from 10 a.m. to 1 p.m. PDT (1 to 4 p.m. EDT) on the day of the eclipse.
Join NASA as a total solar eclipse moves across North America on April 8. Tune in from 10 a.m. to 1 p.m. PDT (1 to 4 p.m. EDT) for live views from across the path, expert commentary, live demos, and more. | Watch on YouTube
What Solar Eclipses Mean for Science
Solar eclipses provide a unique opportunity for scientists to study the Sun and Earth from land, air, and space, plus allow the public to engage in citizen science!
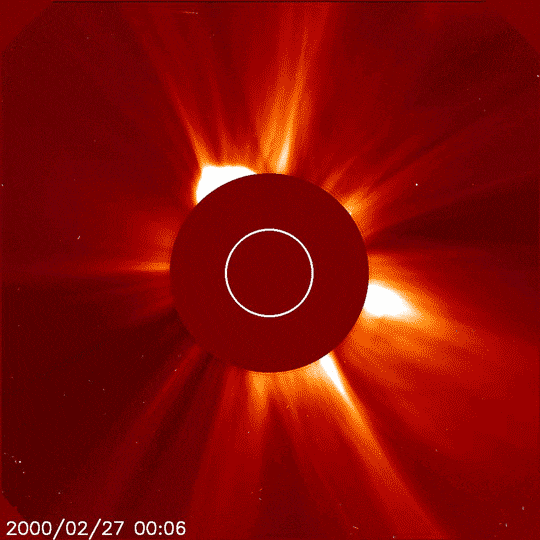
NASA’s Solar and Heliospheric Observatory, or SOHO, constantly observes the outer regions of the Sun’s corona using a coronagraph. Image credit: ESA/NASA/SOHO | + Expand image
Scientists measure incoming solar radiation, also known as insolation, to better understand Earth’s radiation budget – the energy emitted, reflected, and absorbed by our planet. Just as clouds block sunlight and reduce insolation, eclipses create a similar phenomenon, providing a great opportunity to study how increased cloud cover can impact weather and climate.
Solar eclipses can also help scientists study solar radiation in general and the structure of the Sun. On a typical day, the bright surface of the Sun, called the photosphere, is the only part of the Sun we can see. During a total solar eclipse, the photosphere is completely blocked by the Moon, leaving the outer atmosphere of the Sun (corona) and the thin lower atmosphere (chromosphere) visible. Studying these regions of the Sun’s atmosphere can help scientists understand solar radiation, why the corona is hotter than the photosphere, and the process by which the Sun sends a steady stream of material and radiation into space. Annular solar eclipses provide opportunities for scientists to practice their observation methods so that they'll be ready when a total solar eclipse comes around.
Citizen scientists can get involved in collecting data and participating in the scientific process during the eclipse through NASA’s GLOBE program. Anyone in the path of the eclipse and in partial eclipse areas can act as citizen scientists by measuring temperature and cloud cover data and report it using the GLOBE Observer app to help further the study of how eclipses affect Earth’s atmosphere.
Visit NASA's Eclipse Science page to learn more about the many ways scientists are using the eclipse to improve their understanding of Earth, the Moon, and the Sun.
Taking Eclipse Science Farther
Eclipses also make a great jumping-off point to concepts and techniques used in astrophysics and our search for planets beyond our solar system.
Similar to a solar eclipse, a transit occurs when a planet crosses in front of the face of a star. From Earth, the planets Venus and Mercury can occasionally be seen transiting in front of the Sun, appearing as small, dark dots. Transits are also useful for detecting exoplanets – distant planets around other stars. When an exoplanet passes in between its star and Earth, we can measure tiny dips in the star's brightness that tell scientists a planet is there even when it’s too small to see.
Another way that eclipse concepts are used for astrophysics is with coronagraphs, mechanisms inside telescopes that block the light from a star. By creating a sort of artificial eclipse, coronagraphs help scientists search for exoplanets by making much dimmer planets orbiting a star easier to see. For example, NASA’s Nancy Grace Roman Telescope, slated for launch later this decade, will use an advanced coronagraph to analyze and directly image planets that orbit other stars. Learn more about the astrophysics involved in eclipses, including the use of gravitational lensing to study background objects, from NASA’s Universe of Learning.
Learn how the coronagraph instrument on the Nancy Grace Roman Telescope will allow the spacecraft to peer at the universe through some of the most sophisticated sunglasses ever designed. | Watch on YouTube
Solar Eclipse Lessons and Projects
Use these standards-aligned lessons, plus related activities and resources, to get your students excited about the eclipse and the science that will be conducted during the eclipse.
- Student Project
How to Make a Pinhole Camera
Learn how to make your very own pinhole camera to safely see a solar eclipse in action from anywhere the eclipse is visible, partial or full!
Subject Science
Grades K-12
Time < 30 mins
- Collection
NASA's Universe of Learning – Eclipse Resources
Explore a curated collection of resources to expand student learning around the eclipse to related astrophysics concepts.
- Lesson
Moon Phases
Students learn about the phases of the Moon by acting them out. In 30 minutes, they will act out one complete, 30-day, Moon cycle.
Subject Science
Grades 1-6
Time 30-60 mins
- Lesson
Model a Solar Eclipse
Students use simple materials to model a partial, annular, and total solar eclipse.
Subject Science
Grades 1-8
Time 30-60 mins
- Lesson
Measuring Solar Energy During an Eclipse
Students use mobile devices to measure the impact a solar eclipse has on the energy received at Earth’s surface.
Subject Math
Grades 4-7
Time 1-2 hrs
- Lesson
Modeling the Earth-Moon System
Students learn about scale models and distance by creating a classroom-size Earth-Moon system.
Subject Science
Grades 6-8
Time 30-60 mins
- Math Problem
Epic Eclipse
Students use the mathematical constant pi to approximate the area of land covered by the Moon’s shadow during the eclipse.
Subject Math
Grades 6-12
Time < 30 mins
- Math Problem
Eclipsing Enigma
Students use pi to figure out how much of the Sun’s disk will be covered by the Moon during an eclipse and whether it’s a total or annular eclipse.
Subject Math
Grades 7-12
Time < 30 mins
- Mobile App
NASA GLOBE Observer App
Students can become citizen scientists and collect data for NASA’s GLOBE Program using this app available for iOS and Android devices.
Explore More
Eclipse Info
- NASA Eclipses Website
- Calendar of Past and Upcoming Eclipses
- Downloadable Eclipse Map
- NASA HEAT Eclipse Training Slide Decks
Eclipse Safety
Interactives
Citizen Science
Facts & Figures
NASA's Universe of Learning materials are based upon work supported by NASA under award number NNX16AC65A to the Space Telescope Science Institute, working in partnership with Caltech/IPAC, Center for Astrophysics | Harvard & Smithsonian, and the Jet Propulsion Laboratory.
TAGS: Solar Eclipse, Eclipse, Annular Eclipse, K-12 Education, Lessons, Classroom Resources, STEM Resources
Teachable Moments | January 29, 2024
The NASA Cat Video Explained
Find out how the now famous video beamed from space, showing a cat chasing a laser, marked a milestone for space exploration, and find resources to engage students in related STEM learning.
You may have seen in the news last month that NASA beamed a cat video from space. It was all part of a test of new technology known as Deep Space Optical Communications. While the video went down in cat video history, the NASA technology used to transmit the first ultra-high-definition video from deep space also represented a historic advancement for space exploration – the potential to stream videos from the Moon, Mars, and beyond.
Read on to learn how this new technology will revolutionize space communications. Then, explore STEM learning resources that will get students using coding, math, and engineering to explore more about how NASA communicates with spacecraft.
Why did NASA beam a cat video from space?
Communicating with spacecraft across the solar system means sending data – such as commands, images, measurements, and status reports – over enormous distances, with travel times limited by the speed of light. NASA spacecraft have traditionally used radio signals to transmit information to Earth via the Deep Space Network, or DSN. The DSN is made up of an array of giant antennas situated around the globe (in California, Spain, and Australia) that allow us to keep in contact with distant spacecraft as Earth rotates.
When scientists and engineers want to send commands to a spacecraft in deep space, they turn to the Deep Space Network, NASA’s international array of giant antennas. | Watch on YouTube
Although sending transmissions using radio frequencies works well, advances in spacecraft technology mean we're collecting and transmitting a lot more data than in the past. The more data a spacecraft collects and needs to transmit to Earth, the more time it takes to transmit that data. And with so many spacecraft waiting to take their turn transmitting via the DSN's antennas, a sort of data traffic jam is on the horizon.
This interactive shows a real-time simulated view of communications between spacecraft and the DSN. Explore more on DSN Now
To alleviate the potential traffic jam, NASA is testing technology known as optical communications, which allows spacecraft to send and receive data at a higher information rate so that each transmission takes less of the DSN’s time.
The technology benefits scientists and engineers – or anyone who is fascinated by space – by allowing robotic spacecraft exploring planets we can't yet visit in person to send high-definition imagery and stream video to Earth for further study. Optical communications could also play an important role in upcoming human missions to the Moon and eventually to Mars, which will require a lot of data transmission, including video communication.
But why transmit a video of a cat? For a test of this kind, engineers would normally send randomly generated test data. But, in this case, to mark what was a significant event for the project, the team at NASA's Jet Propulsion Laboratory worked with the center's DesignLab to create a fun video featuring the pet of a JPL employee – a now famous orange tabby named Taters – chasing a laser. The video was also a nod to the project's use of lasers (more on that in a minute) and the first television test broadcast in 1928 that featured a statue of the cartoon character Felix the Cat.
This 15-second ultra-high-definition video featuring a cat named Taters was streamed via laser from deep space by NASA on Dec. 11, 2023. | Watch on YouTube
How lasers improve spacecraft communications
The NASA project designed to test this new technology is known as Deep Space Optical Communications, or DSOC. It aims to prove that we can indeed transmit data from deep space at a higher information rate.
To improve upon the rate at which data flows between spacecraft and antennas on Earth, DSOC uses laser signals rather than the radio signals currently used to transmit data. Radio signals and laser signals are both part of the electromagnetic spectrum and travel at the same speed – the speed of light – but they have different wavelengths. The DSOC lasers transmit data in the near-infrared portion of the electromagnetic spectrum, so their wavelength is shorter than radio waves, and they have a higher frequency.
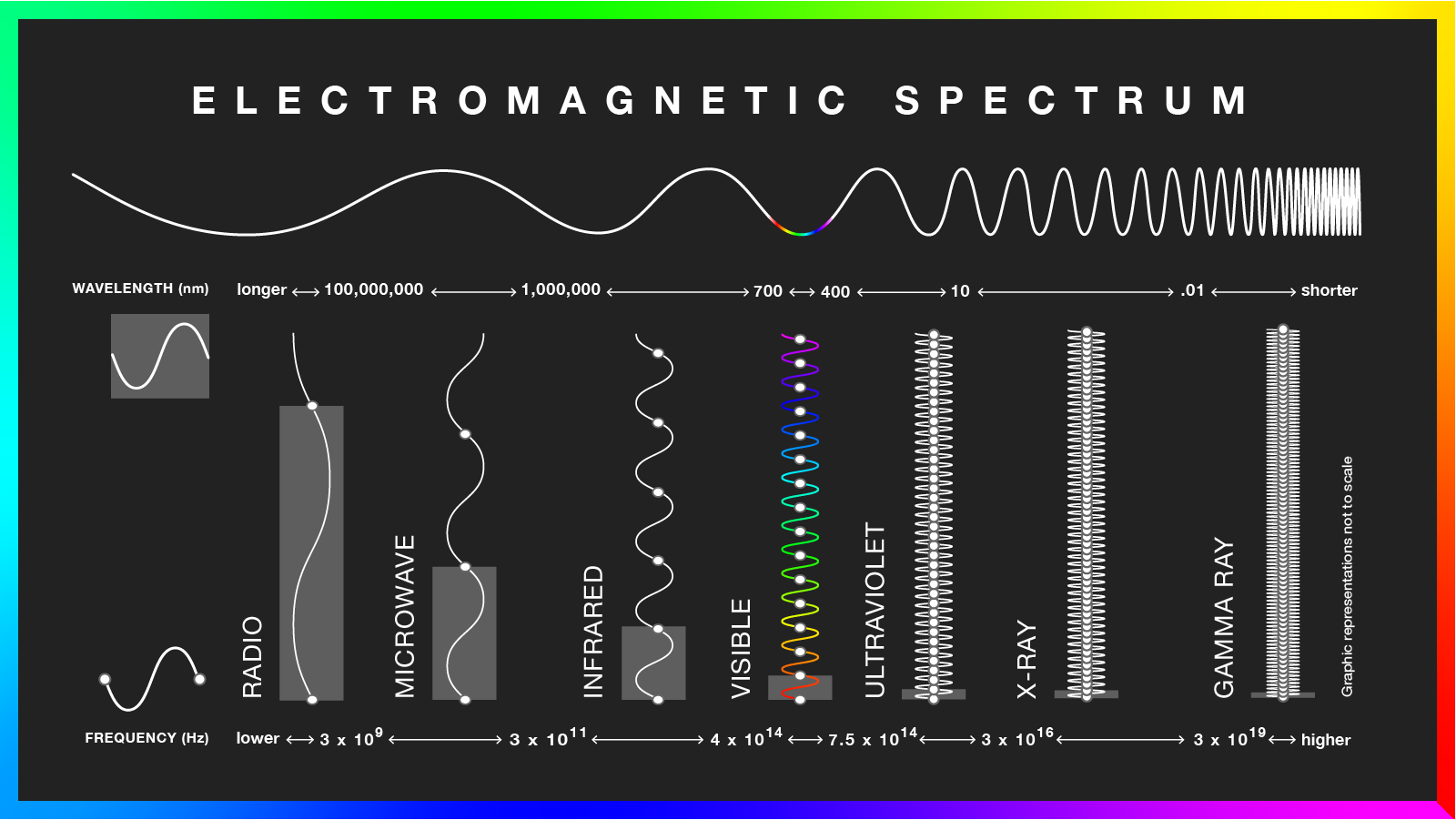
This chart compares the wavelength and frequency range of each kind of wave on the electromagnetic spectrum. Note: The graphic representations are not to scale. Image credit: NASA/JPL-Caltech | + Expand image | › Download low-ink version for printing
Since there are more infrared than radio wavelengths over a particular distance, more data can be sent over the same distance using infrared. And since the speed of infrared and radio waves is equal to the speed of light, this also means that more data can be sent in the same length of time using infrared.
As a result, DSOC’s maximum information rate is around 267 megabits per second (Mbps), faster than many terrestrial internet signals. At that high data rate, the 153.6 megabit cat video took only 0.58 seconds to transmit and another 101 seconds to travel the 19 million miles to Earth at the speed of light. Instead, if we had sent the cat video using Psyche's radio transmitter, which has a data rate of 360 kilobits per second, it would have taken 426 seconds to transmit the video, plus the same speed-of-light travel time, to get to Earth.
Here's how DSOC aims to revolutionize deep space communications. | Watch on YouTube
This kind of spacecraft communications isn't without its challenges. Accurately pointing the narrow laser beam is one of the greatest challenges of optical communications.
DSOC consists of a "flight laser transceiver" aboard the Psyche spacecraft – which is currently on its journey to study the asteroid 16-Psyche – and a receiving station on Earth. The flight transceiver is a 22-centimeter-diameter apparatus that can both transmit and receive signals. Its maximum transmitter strength is a low 4 Watts. For the December 2023 test, a 160-Watt beacon signal was transmitted to the DSOC flight transceiver by a 1-meter telescope located at JPL's Table Mountain facility near Wrightwood, California. This beacon signal was used by the Psyche spacecraft as a pointing reference so it could accurately aim the DSOC transceiver at the Earth receiving station – the 5-meter Hale telescope at Caltech’s Palomar Observatory near San Diego.
This animation shows how DSOC's laser signals are sent between the Psyche spacecraft and ground stations on Earth - first as a pointing reference to ensure accurate aiming of the narrow laser signal and then as a data transmission to the receiving station. | Watch on YouTube
When the DSOC laser beam encounters Earth, it is much narrower than a radio signal transmitted from the same distance. In fact, the laser beam is only a few hundred kilometers wide when it reaches Earth, in sharp contrast with an approximately 2.5-million-kilometer-wide radio signal. This narrow beam must be pointed accurately enough so it not only intersects Earth, but also overlaps the receiving station. To ensure that the beam will be received at Palomar Observatory, the transmission must be aimed not directly at Earth, but at a point where Earth will be in its orbit when the signal arrives after traveling the great distance from the spacecraft.
What's next for laser communications
Engineers will do additional tests of the DSOC system as the Psyche spacecraft continues its 2.2-billion-mile (3.6-billion-kilometer) journey to its destination in the asteroid belt beyond Mars. Over the next couple of years, DSOC will make weekly contacts with Earth. Visit NASA's DSOC website to follow along as NASA puts the system through its paces to potentially usher in a new means of transmitting data through space.
How does the cat video relate to STEM learning?
The DSOC project provides a wonderful opportunity to help students understand the electromagnetic spectrum and learn about real-world applications of STEM in deep space communications. Try out these lessons and resources to get students engaged.
Educator Resources
- Collection
DSOC Lessons for Educators
Get students learning more about optical communications with these standards-aligned coding, engineering, and math lessons.
- Collection
Space Communications Lessons for Educators
These standards-aligned lessons get students using math and engineering to learn how NASA communicates with distant spacecraft.
- Collection
Light and Optics Lessons for Educators
Explore our collection of standards-aligned STEM lessons all about light and optics.
- Teachable Moment
Asteroid Mission Aims to Explore Mysteries of Earth's Core
Explore how NASA's Psyche mission aims to help scientists answer questions about Earth and the formation of our solar system. Then, make connections to STEM learning in the classroom.
- Expert Talk
Teaching Space With NASA – Engineering the Deep Space Network
NASA experts talk about the system of antennas that make up the Deep Space Network and how it's used to communicate with distant spacecraft and collect science.
- Expert Talk
Teaching Space With NASA en Español – La Red del Espacio Profundo de NASA
Un bosquejo de su importancia para la exploración del universo; un esfuerzo en busca de descubrimientos beneficiosos para la humanidad.
Student Resources
- Collection
Space Communications Activities for Students
Explore videos and projects for students about the ways NASA communicates with distant spacecraft.
- Game
Play DSN Uplink-Downlink
In this game you’ll use these big antennas to send information to — and receive information from — NASA’s robotic explorers in the solar system and beyond.
- Student Article
How Does NASA Communicate With Spacecraft?
Get the answer in this article from NASA Space Place.
Explore More
Multimedia
- Image: Explaining the First Ultra-HD Video Sent From Deep Space via Laser
- Public Talk: A Day in the Life of the Deep Space Network
- Infographic: NASA Deep Space Network: Celebrating 50 Years of Communication and Discovery
- JPL Space Images: Deep Space Network
Interactives
Downloads
Websites
- NASA: Deep Space Optical Communications (DSOC)
- NASA: Deep Space Network (DSN)
- NASA Science: Psyche Mission
Articles
- JPL News: NASA's Tech Demo Streams First Video from Deep Space via Laser
- JPL News: 5 Things to Know About NASA's Deep Space Optical Communications
- JPL News: Deep Space Network
TAGS: K-12 Education, Educators, Students, Learning Resources, Teaching Resources, DSOC, DSN, Deep Space Network
Edu News | January 16, 2024
Doing the Math on Why We Have Leap Day
Leap day, Feb. 29, happens every four years because of a mismatch between the calendar year and Earth's orbit. Learn how it works, and get students engaged in leap day STEM.
You may have noticed that there's an extra day on your calendar this year. That's not a typo – it's leap day! Leap day is another name for Feb. 29, a date that typically comes around every four years, during a leap year.
Why doesn't Feb. 29 appear on the calendar every year?
The length of a year is based on how long it takes a planet to revolve around the Sun. Earth takes about 365.2422 days to make one revolution around the Sun. That's about six hours longer than the 365 days that we typically include in a calendar year. As a result, every four years, we have about 24 extra hours that we add to the calendar at the end of February in the form of leap day.
Without leap day, the dates of annual events, such as equinoxes and solstices, would slowly shift to later in the year, changing the dates of each season. After only a century without leap day, summer wouldn’t start until mid-July!
But the peculiar adjustments don't end there. If Earth revolved around the Sun in exactly 365 days and six hours, this system of adding a leap day every four years would need no exceptions. However, Earth takes a little less time than that to orbit the Sun. Rounding up and inserting a 24-hour leap day every four years adds about 45 extra minutes to every four-year leap cycle. That adds up to about three days every 400 years. To correct for that, years that are divisible by 100 don't have leap days unless they’re also divisible by 400.
If you do the math, you'll see that the year 2000 was a leap year, but 2100, 2200 and 2300 will not be.
Have students learn more about leap years with this article from NASA's Space Place, then have them do the math for themselves with this leap day problem set. You can also have students write a letter or poem to be opened on the next leap day or get them learning about orbits across the solar system.
And since we've got an extra 24 hours this year, don't forget to take a little time to relax!
Educator Resources
- Problem Set
Leap Day Math
In this problem set, students calculate the difference between the calendar year and Earth's orbital period to determine when leap years occur.
Subject Math
Grades 5-8
Time Less than 30 mins
- Collection
Solar System Scale & Size Lessons
Explore a collection of standards-aligned lessons all about the size and scale of our solar system.
- Educator Guide
Planetary Poetry
Have students write a poem they can open and re-read next leap day!
Subject Science
Grades 2-12
Time 1-2 hrs
Student Resources
- Article
What Is a Leap Year?
Get the answer in this article from NASA Space Place. Plus, learn if other planets have leap years!
- Article
How Long Is a Year on Other Planets?
Get the answer in this article from NASA Space Place.
- Collection
All About the Size and Scale of the Solar System
Learn how big and far away the planets are with these projects and activities.
TAGS: K-12 Education, Math, Leap Day, Leap Year, Events, Space, Educators, Teachers, Parents, Students, STEM, Lessons, Earth Science, Earth
Edu News | July 27, 2023
We Want to Hear From You
Share your feedback in this 5-minute survey to help JPL Education improve our offerings.
We're working on improving our offerings for educators, students, and families, and we want to hear from you!
This 5-minute survey will help us learn about what resources and offerings you use the most and what you'd like to see us do more of or do differently. Plus, we'd love to hear about the ways you're using JPL Education resources and any impact they've had on you.
This survey is completely anonymous, but you do have the option to share your email address if you would like to be contacted about future opportunities to provide feedback.
Related Resources
For K-12 Educators
Explore our collection of nearly 200 STEAM lessons as well as educational explainers of the latest NASA news, expert talks, teaching materials and more.
- Educator Resources
Lessons for Educators
Explore a collection of standards-aligned STEAM lessons featuring the latest NASA missions and science.
- Articles
Teachable Moments
Take a look inside the latest NASA missions and science news and find out how to get students engaged with related STEAM lessons and activities.
- Educator Resources
NGSS Engineering in the Classroom
Learn how the Next Generation Science Standards in engineering are used at JPL and get activities and tips for bringing them into the classroom.
- Expert Talks
Teaching Space With NASA
Hear from experts and education specialists about the latest missions and science happening at NASA and get your questions answered.
- Showcase
NASA Answers: When Am I Ever Going to Use This?
We asked JPL scientists and engineers to share what STEM concepts they learned in school that they still use in their job today.
- Articles
Teacher Feature
Meet real teachers and find out how they are using STEM education resources from JPL to get creative ideas for your classroom.
- Educator Resources
Educator Resource Center
The NASA-JPL Educator Resource Center provides free math and science teaching materials, training workshops and hands-on demonstrations to both formal and informal educators.
- Educator Resources
NASA STEM Engagement
Engage students in STEM with resources, activities, and programs from NASA.
For K-12 Students
Get students exploring space and science on their own with hands-on projects, video tutorials, expert talks, and career guidance.
- Student Resources
Activities for Students
Explore Earth and space with these hands-on projects, slideshows, videos, and more for K-12 students.
- Student Resources
Learning Space With NASA
Explore space and science activities you can do with NASA at home. Find video tutorials, DIY projects, slideshows, games and more!
- Articles
Career Guidance
Get advice from scientists, engineers and educators about what it takes to work in STEM and how to get a foot in the door.
- Activities for Kids
NASA Space Place
Explore articles, games, and activities about space and space exploration for elementary-school-age kids.
- Activities for Kids
NASA Climate Kids
Articles, games, and resources about Earth and our changing planet for elementary-school-age students.
For College Students
Learn about internships and fellowships at JPL and NASA, plus meet current interns, and get tips on how to make your resume stand out.
- Opportunities
JPL Internships and Fellowships
Discover exciting internships and research opportunities at the leading center for robotic exploration of the solar system.
- Article
How to Get an Internship at JPL
Here's everything you need to know about the world of JPL internships, the skills that will help you stand out, and how to get on the right trajectory even before college.
- Articles
Meet JPL Interns
These interns are pushing the boundaries of space exploration and science at the leading center for robotic exploration of the solar system.
- Opportunities
JPL Jobs: Opportunities for Students
Start here to learn more about internship, fellowship, and postdoc opportunities at JPL and how to apply.
- Opportunities
NASA Internships
Learn about internship opportunities at NASA centers across the U.S., and apply today!
- Articles
NASA Intern Stories
Meet NASA interns and learn about their trajectories to the world of space exploration.
Engage With Us
From student challenges to virtual tours, expert talks, and beyond, there are numerous ways to join the conversation with us.
- Student Challenge
Mission to Mars Student Challenge
Get K-12 students exploring Mars with NASA scientists, engineers, and the Perseverance rover as they learn all about STEM and design their very own mission to the Red Planet!
- Events
JPL Education Events
Explore educational events, workshops and competitions hosted by NASA-JPL online and in Pasadena, California.
- Interactive
JPL Virtual Tour
From visiting mission control to seeing where space robots are built, this interactive tour lets online users explore the historic space facility from anywhere in the world.
- Expert Talks
JPL Lecture Series
This free monthly lecture series at JPL in Pasadena, California – and also available via webcast – features scientists and engineers discussing NASA missions and robotics.
- Competitions
Student Team Competitions
Each year JPL encourages the academic growth and development of local high school students by sponsoring challenging academic competitions in the areas of math, science, and engineering.
- Student Challenge
Artemis Student Challenges
This series of challenges tasks young innovators in middle school, high school, undergraduate and graduate programs to build foundational knowledge on topics and technologies critical to the success of future missions to the Moon and beyond.
Explore More
- Newsletter: JPL Education Updates
- Interactive: NASA Eyes
- Articles: People of NASA
- Events: Request a JPL Speaker
TAGS: K-12 Education, Informal Education, Resources
Teachable Moments | July 24, 2023
Exploring the Mystery of Our Expanding Universe
Learn about a new mission seeking to understand some of the greatest mysteries of our universe, and explore hands-on teaching resources that bring it all down to Earth.
Scientists may soon uncover new insights about some of the most mysterious phenomena in our universe with the help of the newly launched Euclid mission. Built and managed by the European Space Agency, Euclid will use a suite of instruments developed, in part, by NASA's Jet Propulsion Laboratory to explore the curious nature of dark energy and dark matter along with their role in the expansion and acceleration of our universe.
Read on to learn how the Euclid mission will probe these cosmological mysteries. Then, find out how to use demonstrations and models to help learners grasp these big ideas.
Why It’s Important
No greater question in our universe promotes wonder in scientists and non-scientists alike than that of the origin of our universe. The Euclid mission will allow scientists to study the nearly imperceptible cosmic components that may hold exciting answers to this question.
Edwin Hubble's observations of the expanding universe in the 1920s marked the beginnings of what's now known as the big-bang theory. We've since made monumental strides in determining when and how the big bang would have taken place by looking at what's known as cosmic background radiation using instruments such as COBE and WMAP in 1989 and 2001, respectively. However, there's one piece of Hubble's discovery that still has scientists stumped: our universe is not only expanding, but as scientists discovered in 1998, that expansion is also accelerating.
This side by side comparison shows a constant rate of expansion of the universe, represented by the expanding sphere on the left, and an accelerating rate of expansion of the universe, represented by the expanding sphere on the right. Each dot on the spheres represents a galaxy and shows how galaxies move apart from each other faster in the universe that has an accelerating rate of expansion. | Watch on YouTube
How can this be? It makes intuitive sense that, regardless of the immense force of the big bang that launched all matter across the known universe 13.8 billion years ago, that matter would eventually come to a rest and possibly even start to collapse. Instead, it's as if we've dropped a glass onto the ground and discovered that the shards are flying away from us faster and faster into perpetuity.
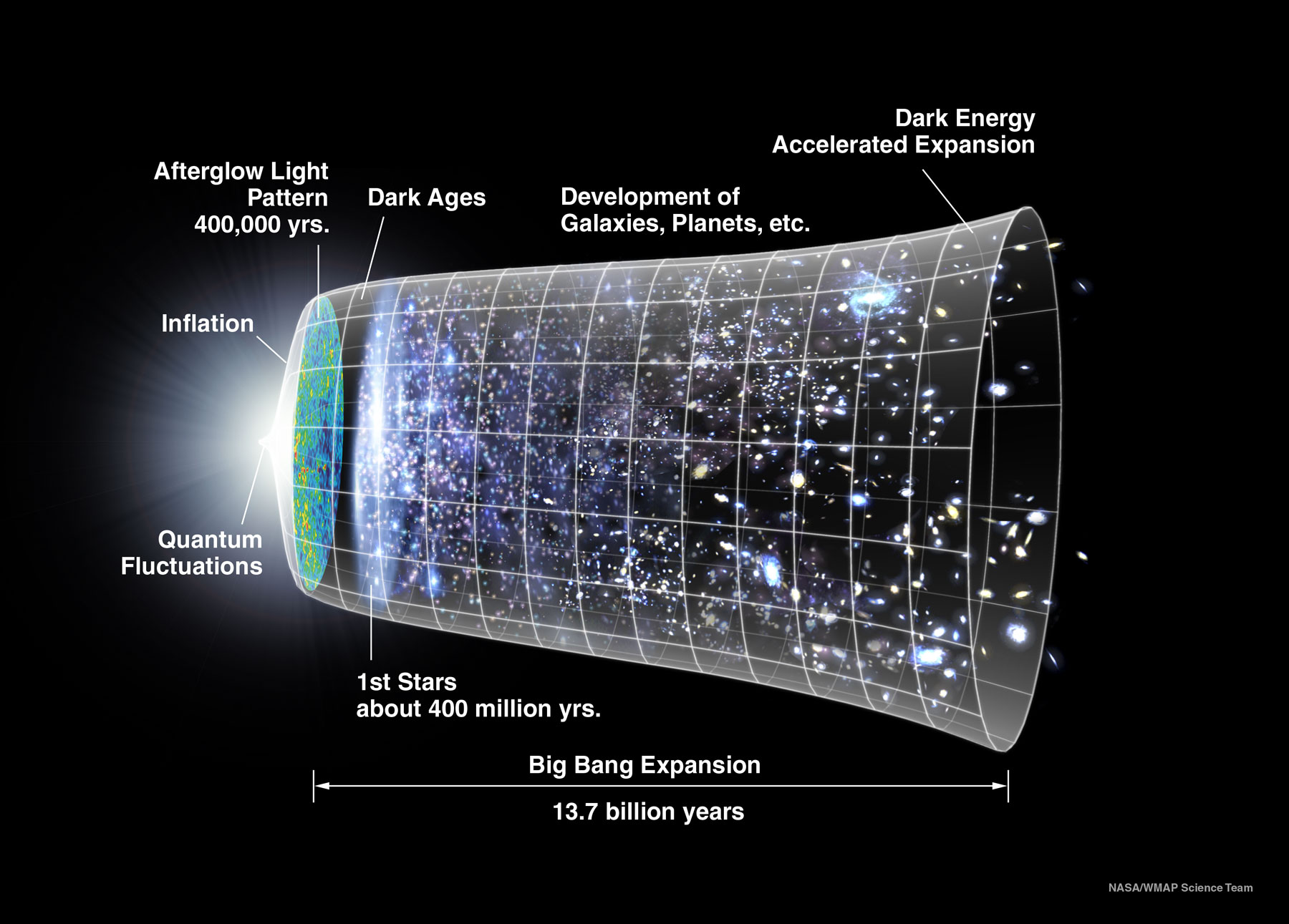
An illustrated timeline of the universe. Credit: WMAP | + Expand image
Scientists believe that answers may lie in two yet-to-be-understood factors of our universe: dark matter and dark energy. Dark matter is unlike the known matter we experience here on Earth, such as what's found on the periodic table. We can't actually see dark matter; we can only infer its presence. It has mass and therefore gravity, making it an attractive force capable of pulling things together. Amazingly, dark matter makes up roughly 27% of the known universe compared with the much more modest 5% of "normal matter" that we experience day to day. However, dark matter is extremely dilute throughout the universe with concentrations of 105 particles per cubic meter.
This animated pie chart shows rounded values for the three known components of the universe: visible matter (5%), dark matter (27%), and dark energy (68%). Credit: NASA's Goddard Space Flight Center | › Full video and caption
In opposition to the attractive force of dark matter, we have dark energy. Dark energy is a repulsive force and makes up roughly 68% of energy in the known universe. Scientists believe that the existence of dark energy and the amount of repulsion it displays compared with dark matter is what's causing our universe to not only expand, but also to expand faster and faster.
Dr. Jennifer Wiseman, a senior project scientist with the Hubble Space Telescope mission, explains how the mission has been helping scientists learn more about dark energy. Credit: NASA Goddard | Watch on YouTube
But to truly understand this mysterious force and how it interacts with both dark matter and normal matter, scientists will have to map barely detectable distortions of light traversing the universe, carefully measuring how that light changes over time and distance in every direction. As JPL Astrophysicist Jason Rhodes explains, “Dark energy has such a subtle effect that we need to survey billions of galaxies to adequately map it.”
And that's where Euclid comes in.
How It Works
The European Space Agency and NASA each contributed to the development of the Euclid mission, which launched from Cape Canaveral Space Force Station in Florida on July 1. The spacecraft consists of a 1.2-meter (48-inch) space telescope and two science instruments: an optical camera and a near-infrared camera that also serves as a spectrometer. These instruments will provide a treasure trove of data for scientists of numerous disciplines, ranging from exoplanet hunters to cosmologists.

Light waves get stretched as the universe expands similar to how this ink mark stretches out as the elastic is pulled. Get students modeling and exploring this effect with this standards-aligned math lesson. Credit: NASA/JPL-Caltech | + Expand image
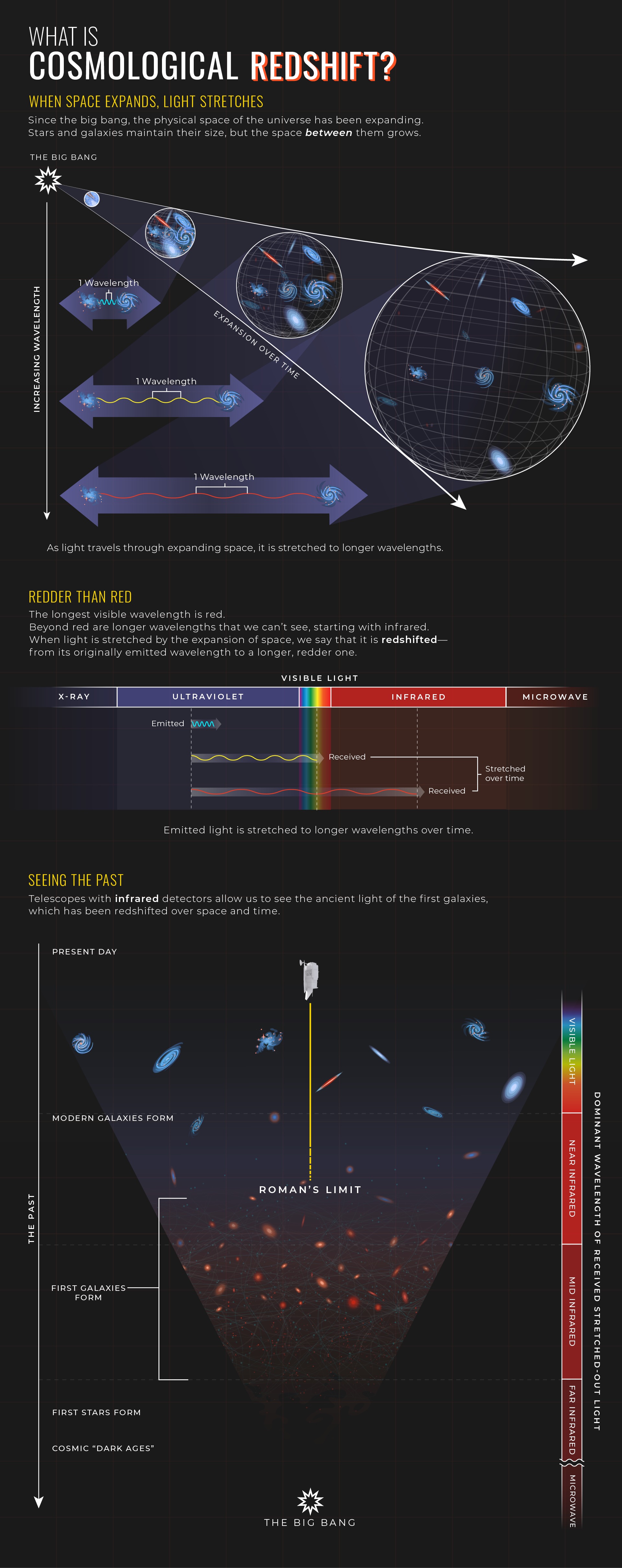
This graphic illustrates how cosmological redshift works and how it offers information about the universe’s evolution. Credit: NASA, ESA, Leah Hustak (STScI) | › Full image and caption
As Gisella de Rosa at the Space Telescope Science Institute explains, “The ancillary science topics we will be able to study with Euclid range from the evolution of the objects we see in the sky today to detecting populations of galaxies and creating catalogs for astronomers. The data will serve the entire space community.”
The cameras aboard Euclid will operate at 530-920 nanometers (optical light) and at 920-2020 nanometers (near infrared) with each boasting more than 576 million and 65 million pixels, respectively. These cameras are capable of measuring the subtle changes to the light collected from celestial objects and can determine the distances to billions of galaxies across a survey of 15,000 square degrees – one-third of the entire sky.
Meanwhile, Euclid's spectrometer will collect even more detailed measurements of the distance to tens of millions of galaxies by looking at redshift. Redshift describes how wavelengths of light change ever so slightly as objects move away from us. It is a critical phenomenon for measuring the speed at which our universe is expanding. Similar to the way sound waves change as a result of the Doppler effect, wavelengths of light are compressed to shorter wavelengths (bluer) as something approaches you and extended to longer wavelengths (redder) as it moves away from you. As determined by a Nobel Prize winning team of astronomers, our universe isn’t just red-shifting over time, distant objects are becoming redder faster.
Euclid will measure these incredibly minuscule changes in wavelength for objects near and far, providing an accurate measurement of how the light has changed as a factor of time and distance and giving us a rate of acceleration of the universe. Furthermore, Euclid will be able to map the relative densities of dark matter and normal matter as they interact with dark energy, creating unevenly distributed pockets of more attractive forces. This will allow scientists to identify minute differences in where the universe is expanding by looking at the way that light is altered or "lensed."
The multi-dimensional maps created by Euclid – which will include depth and time in addition to the height and width of the sky – will inform a complementary mission already in development by NASA, the Nancy Grace Roman Space Telescope. Launching in 2026, this space telescope will look back in time with even greater detail, targeting areas of interest provided by Euclid. The telescope will use instruments with higher sensitivity and spatial resolution to peer deeper into redshifted and faint galaxies, building on the work of Euclid to look farther into the accelerating universe. As Caltech’s Gordon Squires describes it: “We’re trying to understand 90% of our entire universe. Both of these telescopes will provide essential data that will help us start to uncover these colossal mysteries.”
Teach It
The abstract concepts of the scope and origin of our universe and the unimaginable scale of cosmology can be difficult to communicate to learners. However, simple models and simulations can help make these topics more tangible. See below to find out how, plus explore more resources about our expanding universe.Resources
- Educator Guide
Model the Expanding Universe
Students learn about the role of dark energy and dark matter in the expansion of the universe, then make a model using balloons.
Subject Science
Grades 6-12
Time 30-60 mins
- Educator Guide
How Do We See Dark Matter?
Students will make observations of two containers and identify differences in content, justify their claims and make comparisons to dark matter observations.
Subject Science
Grades 6-12
Time Less than 30 mins
- Educator Guide
Math of the Expanding Universe
Students will learn about the expanding universe and the redshift of lightwaves, then perform their own calculations with a distant supernova.
Subject Science
Grades 9-12
Time 30-60 mins
- Collection
ViewSpace: Dark Energy Videos
Use this collection of short videos to introduce learners to the concept of dark energy.
- Collection
ViewSpace: Dark Matter Videos
Use this collection of short videos to introduce learners to the concept of dark matter.
- Science Briefing
Hubble Constant Discrepancies: Implications for Our Expanding Universe
Hear experts discuss how the rate of the universe is measured in different cosmological epochs and what the differences in those measures can tell us.
- Game
Roman Space Observer
How many astrophysical objects can you catch?
Explore More
- Article for Kids: What Is a Supernova?
- Article for Kids: What Is Dark Matter?
- Article: What Is Dark Energy?
- Article: Gravitational Lensing - Shining a Light on Dark Matter
- Facts & Figures: Euclid Mission - NASA
- Facts & Figures: Nancy Grace Roman Space Telescope
NASA's Universe of Learning materials are based upon work supported by NASA under award number NNX16AC65A to the Space Telescope Science Institute, working in partnership with Caltech/IPAC, Center for Astrophysics | Harvard & Smithsonian, and the Jet Propulsion Laboratory.
TAGS: K-12 Education, Teaching, Teachers, Educators, Resources, Universe, Dark Matter, Dark Energy, Euclid, Nancy Grace Roman Space Telescope, Universe of Learning
Teachable Moments | January 3, 2023
How InSight Revealed the Heart of Mars
As NASA retires its InSight Mars lander, here's a look at some of the biggest discoveries from the first mission designed to study the Red Planet's interior – plus, how to make connections to what students are learning now.
After more than four years listening to the “heartbeat” of Mars, NASA is saying goodbye to the InSight lander as the mission on the Red Planet comes to an end. On Dec. 21, 2022 scientists wrapped up the first-of-its-kind mission to study the interior of Mars as dust in the Martian atmosphere and on the spacecraft’s solar panels prevented the lander from generating enough power to continue.
Read on to learn how the mission worked, what it discovered, and how to bring the science and engineering of the mission into the classroom.
How It Worked

The locations of InSight's three main science tools, SEIS, HP3, and RISE are labeled in this illustration of the lander on Mars. | + Expand image | › Full image and caption
The InSight lander was designed to reveal the processes that led to the formation of Mars – as well as Earth, the Moon, and all rocky worlds. This meant meeting two main science goals.
First, scientists wanted to understand how Mars formed and evolved. To do that, they needed to investigate the size and make-up of Mars’ core, the thickness and structure of its crust, the structure of the mantle layer, the warmth of the planet's interior, and the amount of heat flowing through the planet.
Second, to study tectonic activity on Mars, scientists needed to determine the power, frequency, and location of “marsquakes” as well as measure how often meteoroids impacted the Red Planet, creating seismic waves.
Engineers equipped InSight with three main science tools that would allow researchers to answer these questions about Mars.
SEIS, a seismometer like the ones used on Earth to record earthquakes, measured the seismic waves on Mars. These waves, which travel through the Red Planet, can tell scientists a lot about the areas they pass through. They even carry clues about whether it was a marsquake or meteorite impact that created the waves.

InSight captured these images of clouds drifting in the distance, visible just beyond the dome-like top of the SEIS instrument. Credit: NASA/JPL-Caltech | + Expand image | › Full image and caption
InSight's Heat Flow and Physical Properties Package, or HP3, was an instrument designed to burrow 16 feet (five meters) into Mars to measure the temperature at different depths and monitor how heat flowed out toward the surface. However, the self-hammering probe, informally called the "mole," struggled to dig itself in due to the unexpected consistency of the top few inches of Mars regolith at the landing site. Using full-size models of the lander and probe, engineers recreated InSight’s environment here on Earth to see if they could find a solution to the issue. They tested solutions that would allow the probe to penetrate the surface, including pressing the scoop attached to InSight’s robotic arm against the probe. While the effort serves as a great real-world example of how engineers work through problems with distant spacecraft, ultimately, none of the solutions allowed the probe to dig past the surface when attempted on Mars.
In 2019, InSight mission scientist/engineer Troy Hudson shared the game plan for getting the mission's heat probe digging again on Mars. Ultimately, the team wasn't able to to get the "mole" working, but the effort is a great real-world example of how engineers work through problems with distant spacecraft. | Watch on YouTube
InSight’s third experiment, called RISE, used the spacecraft’s radio antennas to precisely measure the lander's position on the surface of Mars. The interior structure of Mars affects the planet’s motion, causing it to wobble. Measuring InSight’s position as the planet wobbled helped scientists gain a better understanding of the core and other layered structures that exist within the interior of Mars.
What We Discovered
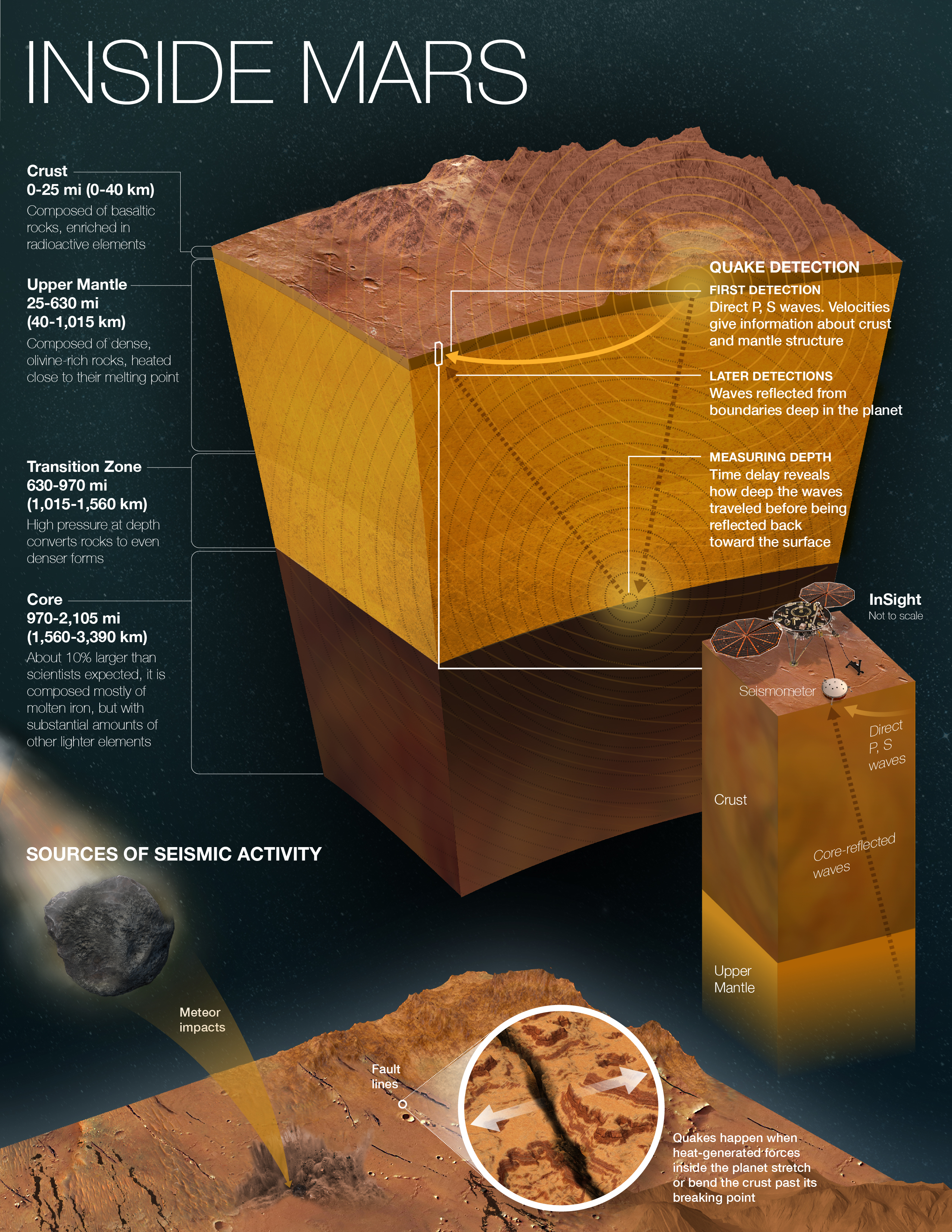
Using its seismometer, InSight gained a deeper understanding of the interior layers of Mars, as detailed in this graphic. Image credit: NASA/JPL-Caltech | + Expand image | › Full image and caption
InSight’s instruments enabled the mission science team to gain an understanding of not only the depth of Mars’ crust, mantle, and core, but also the composition of those features. They also learned just how active Mars really is.
The Structure of Mars
Working our way from the surface to the center of the planet, scientists found Mars’ crust was thinner than expected. Seismic waves detected by SEIS indicate that the crust is made up of three sub-layers, similar to Earth’s crust. The top-most layer of the crust is about six miles (10 kilometers) deep, while the denser layers of the crust, which contain more felsic, or iron-rich, material extend downward to about 25 miles (40 kilometers) below the surface. As seismic waves from a marsquake or a meteorite impact spread across the surface and through the interior of the planet, they can reflect off of underground layers, giving scientists views into the unseen materials below. Measuring how the waves change as a result of these reflections is how scientists unveiled the underground structure of Mars.
Like Earth, Mars has a lithosphere, a rigid layer made up of the crust and upper mantle. The Martian lithosphere extends about 310 miles (500 kilometers) below the surface before it transitions into the remaining mantle layer, which is relatively cool compared with Earth’s mantle. Mars’ mantle extends to 969 miles (1,560 kilometers) below the surface where it meets the planet’s core.

In this lesson from the "Pi in the Sky" math challenge, students use measurements from InSight along with pi to calculate the density of Mars' core. Image credit: NASA/JPL-Caltech | + Expand image | › Go to the lesson
Scientists measured the core of Mars and found it to be larger than expected, with a radius of 1,137 miles (1,830 kilometers). With this information, scientists were able to estimate the density of Mars' core, which turned out to be less dense than anticipated, meaning it contains lighter elements mixed in with iron. Scientists also confirmed that the planet contains a liquid core. While we know that Earth has a liquid outer core and solid inner core, scientists will need to further study the data returned from InSight to know if there is also a solid inner core on Mars.
As scientists continue to study the data returned from InSight, we could learn even more about how Mars formed, how its magnetic field developed, and what materials make up the core, which could ultimately help us better understand how Earth and other planets formed.
Marsquakes
InSight discovered that Mars is a very active planet. A total of 1,319 marsquakes were detected after the SEIS instrument was placed on the surface. The largest, which was estimated to be a magnitude 5, was detected in May of 2022.
Unlike Earth, where the crust is broken into large pieces called plates that continually shift around causing earthquakes, Mars’ crust is made up of one solid plate, somewhat like a shell. However, as the planet cools, the crust shrinks, creating breaks called faults. This breaking action is what causes marsquakes, and the seismic waves generated by the quakes are what help scientists figure out when and where the quakes occurred and how powerful they were.

In this math problem from the "Pi in the Sky" series, students use pi to identify the timing and location of a hypothetical marsquake recorded by InSight. Image credit: NASA/JPL-Caltech | + Expand image | › Go to the lesson
Nearly all of the strongest marsquakes detected by InSight came from a region known as Cerberus Fossae, a volcanic region that may have had lava flows within the past few million years. Volcanic activity, even without lava flowing on the surface, can be another way marsquakes occur. Images from orbiting spacecraft show boulders that have fallen from cliffs in this region, perhaps shaken loose by large marsquakes.

This seismogram shows the largest quake ever detected on another planet. Estimated at magnitude 5, this quake was discovered by InSight on May 4, 2022. Listen to a sonification of this seismogram. | + Expand image | › Full image and caption
Conversely, InSight didn't detect any quakes in the volcanic region known as Tharsis, the home of three of Mars’ largest volcanos that sit approximately one-third of the way around the planet from InSight. This doesn’t necessarily mean the area is not seismically active. Scientists think there may be quakes occurring, but the size of Mars’ liquid core creates what’s known as a shadow zone – an area into which seismic waves don’t pass – at InSight's location.
Meteorite Impacts
On Sept. 5, 2021, InSight detected the impacts of a meteoroid that entered the Martian atmosphere. The meteoroid exploded into at least three pieces that reached the surface and left behind craters. NASA’s Mars Reconnaissance Orbiter passed over the impact sites to capture images of the three new craters and confirm their locations.
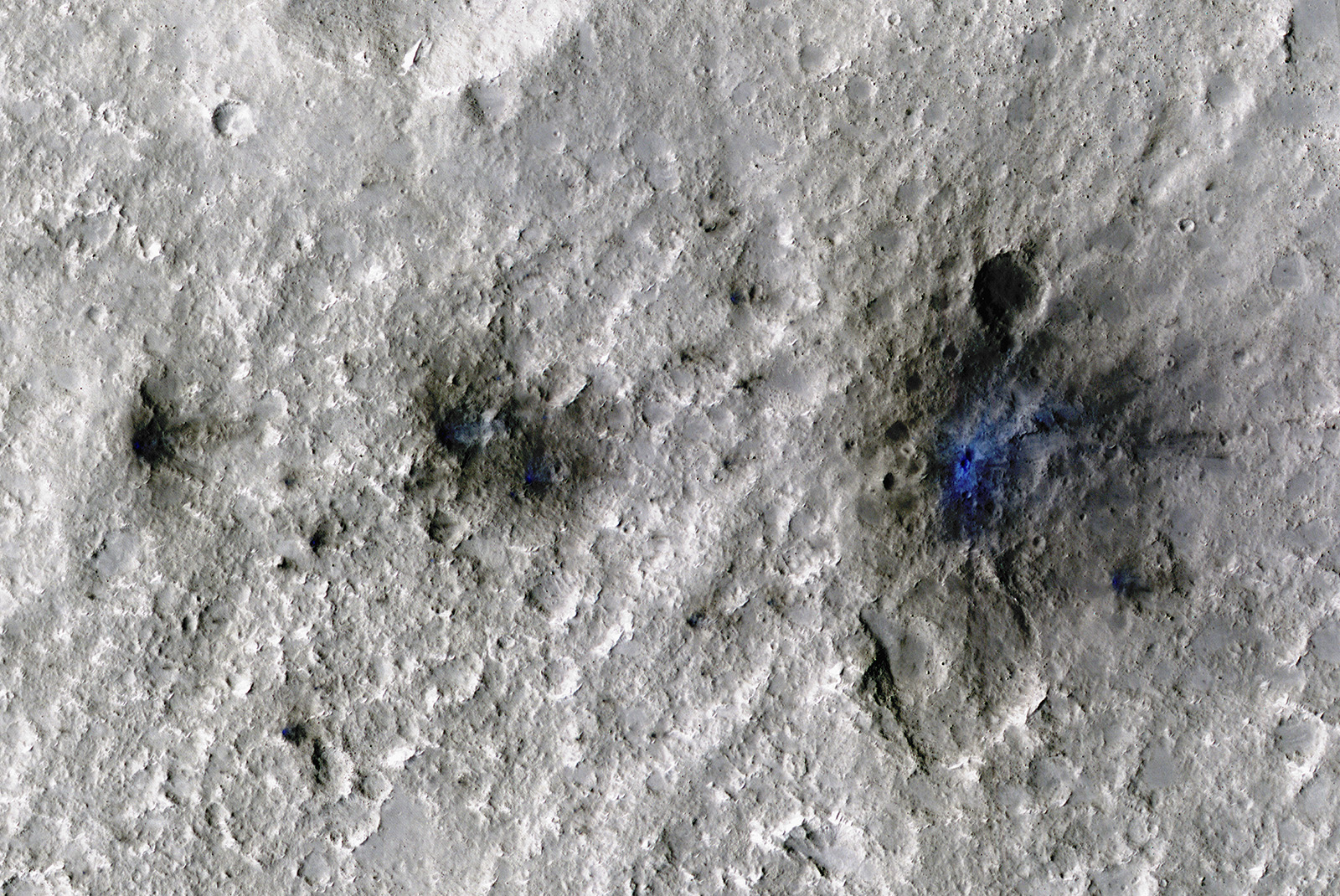
This image, captured by the Mars Reconnaissance Orbiter, shows the craters (in blue) formed by a meteroid impact on Mars on Sept. 5, 2021. The impact was the first to be detected by InSight. Image credit: NASA/JPL-Caltech/University of Arizona | + Expand image | › Full image and caption
“After three years of waiting for an impact, those craters looked beautiful,” said Ingrid Daubar of Brown University, a Mars impacts specialist.
Mars’ thin atmosphere, which is less than 1% as dense as Earth’s, means meteoroids have a better chance of not disintegrating in the heat and pressure that builds up as they pass through the atmosphere to the planet’s surface. Despite this fact and Mars' proximity to the asteroid belt, the planet proved to be a challenging location to detect meteorite impacts because of "noise" in the data created by winds blowing on SEIS and seasonal changes in the atmosphere.
With the confirmation of the September 2021 impacts, scientists were able to identify a telltale seismic signature to these meteorite impacts. With this information in hand, they looked back through InSight's data and found three more impacts – one in 2020 and two in 2021. Scientists anticipate finding even more impacts in the existing data that might have been hidden by the noise in the data.
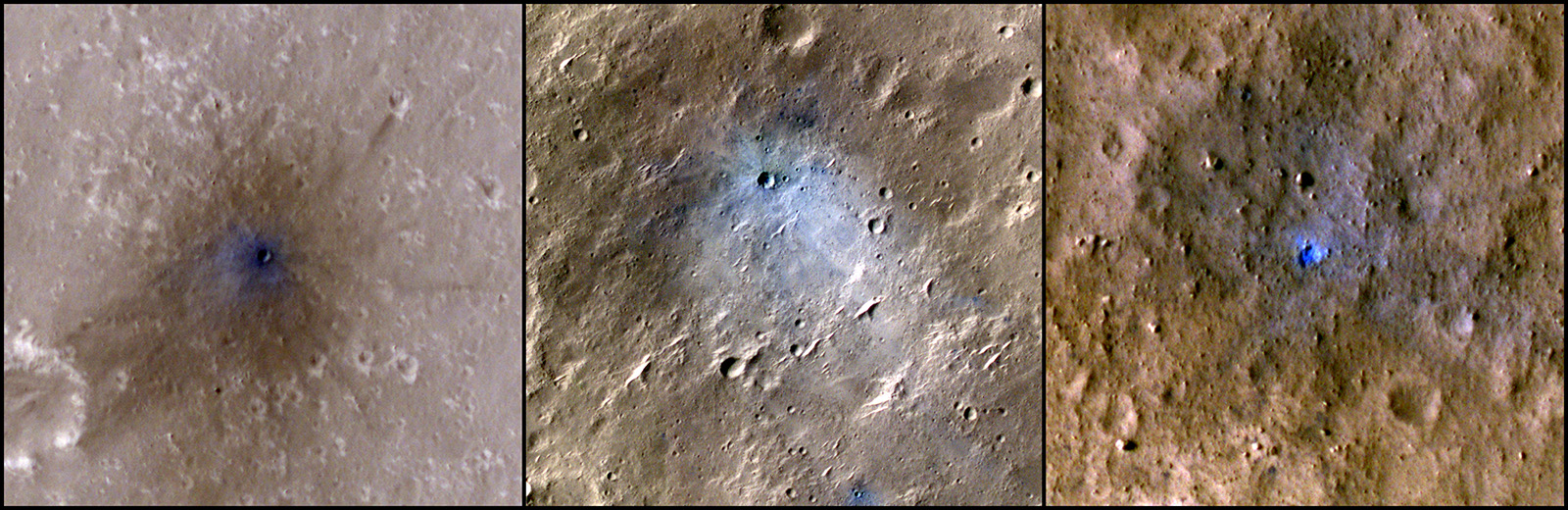
This collage shows three other meteoroid impacts on Mars that were detected by the seismometer on InSight and captured by the Mars Reconnaissance Orbiter. Image credit: Credit: NASA/JPL-Caltech/University of Arizona | + Expand image | › Full image and caption
Meteorite impacts are an invaluable piece of understanding the planet’s surface. On a planet like Earth, wind, rain, snow and ice wear down surface features in a process known as weathering. Plate tectonics and active volcanism refresh Earth’s surface regularly. Mars’ surface is older and doesn't go through those same processes, so a record of past geologic events like meteorite impacts is more apparent on the planet's surface. By counting impact craters visible on Mars today, scientists can update their models and better estimate the number of impacts that occurred in the early solar system. This gives them an improved approximation of the age of the planet’s surface.
Learn how InSight detected the first seismic waves from a meteoroid on Mars and how the lander captured the sound of the space rock striking the surface. | Watch on YouTube
Why It's Important
Before InSight touched down, all Mars missions – landers, rovers, orbiters and flyby spacecraft – studied the surface and atmosphere of the planet. InSight was the first mission to study the deep interior of Mars.
Even with the InSight mission drawing to a close, the science and engineering of the mission will continue to inform our understanding of the Red Planet and our solar system for years as researchers further examine the data returned to Earth. Keep up to date with the latest findings from InSight scientists and engineers on the mission website.
Teach It
Explore these lessons in geology, physics, math, coding and engineering to connect student learning to the InSight mission and the real-world STEM that happens at NASA.
Educator Resources
- Collection
InSight Lessons for Educators
Explore a collection of standards-aligned lessons to bring the science and engineering of the InSight mission into the classroom.
- Collection
NASA's Mission to Mars Student Challenge
Get K-12 students exploring Mars with NASA scientists, engineers, and the Perseverance rover as they learn all about STEM and design their very own mission to the Red Planet!
- Teachable Moments
NASA InSight Lander to Get First Look at ‘Heart’ of Mars
Learn what it takes to travel to Mars and get students engaged with lessons in calculating trajectories, plus building and launching rockets.
- Teachable Moments
Mars Landing to Deliver Science Firsts
Find out how NASA’s InSight lander will collect all-new science at Mars, then get students doing similar investigations in the classroom.
Student Activities
Explore More
- Website: Mars InSight Mission
- Podcast: On a Mission - Season 1
- Articles: JPL News - InSight Mission
- Videos: InSight Mission Videos
- Images: InSight Mission Images
- Video: Interns Explore the Future at NASA-JPL
- Videos: Inside InSight - YouTube Playlist
- Videos: InSight Mission to Mars - YouTube Playlist
- Interactive: Experience InSight
- Website: NASA Mars Exploration
- Articles: People - Meet the Martians
- Resources for Kids: Space Place - All About Mars
TAGS: K-12 Education, Classrooms, Teaching, Teachers, Resources, Teachable Moments, Mars, InSight, Missions, Spacecraft, Marsquakes
Teachable Moments | December 8, 2022
NASA Mission Takes a Deep Dive Into Earth's Surface Water
Explore how and why the SWOT mission will take stock of Earth's water budget, what it could mean for assessing climate change, and how to bring it all to students.
Update: Dec. 15, 2022 – NASA, the French space agency, and SpaceX are now targeting 3:46 a.m. PST (6:46 a.m. EST) on Friday, Dec.16, for the launch of the Surface Water and Ocean Topography (SWOT) satellite. Visit NASA's SWOT launch blog for the latest updates.
NASA is launching an Earth-orbiting mission that will map the planet’s surface water resources better than ever before. Scheduled to launch on Dec. 16 from Vandenberg Space Force Base in California, the Surface Water and Ocean Topography, or SWOT mission is the latest international collaboration designed to monitor and report on our home planet. By providing us with a highly detailed 3D view of rivers, lakes, and oceans, SWOT promises to improve our understanding of Earth’s water cycle and the role oceans play in climate change, as well as help us better respond to drought and flooding.
Read on to find out why we're hoping to learn more about Earth's surface water, get to know the science behind SWOT's unique design, and follow along with STEM teaching and learning resources.
Why It's Important
Observing Earth from space provides scientists with a global view that is important for understanding the whole climate system. In the case of SWOT, we will be able to monitor Earth’s surface water with unprecedented detail and accuracy. SWOT will provide scientists with measurements of water volume change and movement that will inform our understanding of fresh water availability, flood hazards, and the mechanisms of climate change.
Scientists and engineers provide an overview of the SWOT mission. Credit: NASA/JPL-Caltech | Watch on YouTube
Water Flow
Scientists use a variety of methods to track Earth’s water. These include stream and lake gauges and even measurements from space such as sea surface altimetry and gravitational measurements of aquifer volumes. Monitoring of river flow and lake volume is important because it can tell us how much freshwater is readily available and at what locations. River flow monitoring can also help us make inferences about the downstream environmental impact. But monitoring Earth’s surface water in great detail with enough frequency to track water movement has proven challenging. Until now, most monitoring of river flow and lake levels has relied on water-flow and water-level gauges placed across Earth, which requires that they be accessible and maintained. Not all streams and lakes have gauges and previous space-based altimetry and gravitational measurements, though useful for large bodies of water, have not been able to adequately track the constant movement of water through smaller rivers or lakes.
Here's why understanding Earth’s "water budget" is an important part of understanding our planet and planning for future water needs.
SWOT will be able to capture these measurements across the globe in 3D every 21 days. The mission will monitor how much water is flowing through hundreds of thousands of rivers wider than 330 feet (100 meters) and keep a close watch on the levels of more than a million lakes larger than 15 acres (6 hectares). Data from the mission will be used to create detailed maps of rivers, lakes, and reservoirs that will enable accurate monitoring to provide a view of freshwater resources that is not reliant on physical access. Meanwhile, SWOT’s volumetric measurements of rivers, lakes, and reservoirs will help hydrologists better track drought and flooding impacts in near-real-time.
Coastal Sea Level Rise
SWOT will measure our oceans with unprecedented accuracy, revealing details of ocean features as small as 9 miles (15 kilometers) across. SWOT will also monitor sea levels and tides. Though we have excellent global sea level data, we do not have detailed sea level measurements near coastlines. Coastal sea levels vary across the globe as a result of ocean currents, weather patterns, land changes, and other factors. Sea levels are rising faster than ever, and higher sea levels also mean that hurricane storm surges will reach farther inland than ever before, causing substantially more damage than the same category of hurricanes in the past. SWOT will be able to monitor coastal sea level variations and fill gaps in the observations we currently have from other sources.
What is sea level rise and what does it mean for our planet? | › View Transcript
Ocean Heat Sinks
Further contributing to our understanding of the role Earth’s oceans play in climate change, SWOT will explore how the ocean absorbs atmospheric heat and carbon, moderating global temperatures and climate change. Scientists understand ocean circulation on a large scale and know that ocean currents are driven by temperature and salinity differences. However, scientists do not currently have a good understanding of fine-scale ocean currents, where most of the ocean's motion-related energy is stored and lost. Circulation at these fine scales is thought to be responsible for transporting half of the heat and carbon from the upper ocean to deeper layers. Such downward ocean currents have helped to mitigate the decades-long rise in global air temperatures by absorbing and storing heat and carbon away from the atmosphere. Knowing more about this process is critical for understanding the mechanisms of global climate change.
JPL scientist Josh Willis uses a water balloon to show how Earth's oceans are absorbing most of the heat being trapped on our warming world. | › Related lesson
These fine-scale ocean currents also transport nutrients to marine life and circulate pollutants such as crude oil and debris. Understanding nutrient transport helps oceanographers assess ocean health and the productivity of fisheries. And tracking pollutants aids in natural hazard assessment, prediction, and response.
How It Works
A joint effort between NASA and the French space agency – with contributions from the Canadian and UK space agencies – SWOT will continue NASA’s decades-long record of monitoring sea surface height across the globe. But this mission will add a level of detail never before achieved.
SWOT will measure more than 90% of Earth’s surface water, scanning the planet between 78°N latitude and 78°S latitude within 1 centimeter of accuracy and retracing the same path every 21 days. Achieving this level of accuracy from a spacecraft height of 554 miles (891 kilometers) requires that the boom using radar to measure water elevation remain stable within 2 microns – or about 3% of the thickness of a human hair.
This visualization shows ocean surface currents around the world during the period from June 2005 through December 2007. With its new, high resolution wide-swath measurements, SWOT will be able to observe eddies and current features at greater resolution than previously possible. Credit: NASA Scientific Visualization Studio | Watch on YouTube
Prior to SWOT, spacecraft have used conventional nadir, or straight-down, altimetry to measure sea surface height. Conventional nadir altimetry sends a series of radar or laser pulses down to the surface and measures the time it takes for each signal to return to the spacecraft, thus revealing distances to surface features. To acquire more detailed information on surface water, SWOT will use an innovative instrument called the Ka-band Radar Interferometer, or KaRIn, to measure water height with exceptional accuracy. Ka-band is a portion of the microwave part of the electromagnetic spectrum. SWOT uses microwaves because they can penetrate clouds to return data about water surfaces.
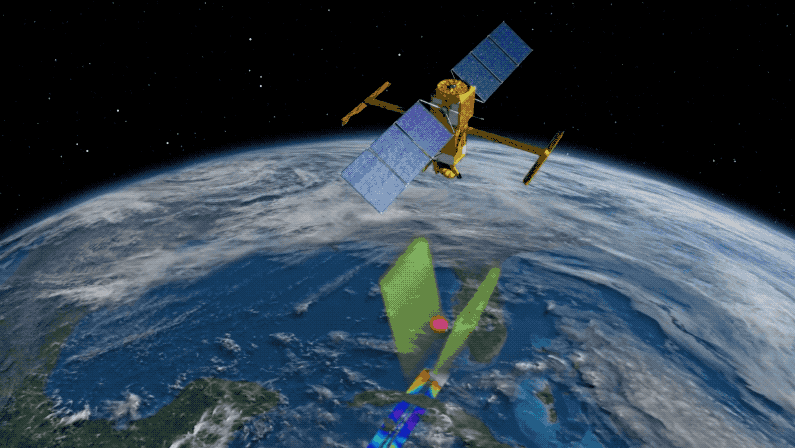
SWOT will track Earth's surface water in incredible detail using an innovative instrument called the Ka-band Radar Interferometer, or KaRIn. Image credit: NASA/JPL-Caltech | + Expand image
The KaRIn instrument uses the principles of synthetic aperture radar combined with interferometry to measure sea surface height. A radar signal is emitted from the end of the 10-meter-wide boom on the spacecraft. The reflected signal is then received by antennas on both ends of the boom, capturing data from two 30-mile (50-kilometer) wide swaths on either side of the spacecraft. The received signals will be slightly out of sync, or phase, from one another because they will travel different distances to return to the receivers on either end of the boom. Knowing the phase difference, the distance between the antennas, and the radar wavelength allows us to calculate the distance to the surface.

Radar signals bounced off the water’s surface will be received by antennas on both ends of SWOT's 10-meter-wide boom. The received signals will be slightly out of phase because they will travel different distances as they return to the receivers. Scientists use this phase difference and the radar wavelength to calculate the distance to the surface. Image credit: NASA/JPL-Caltech | + Expand image
The observations acquired by the two antennas can be combined into what is known as an interferogram. An interferogram is a pattern of wave interference that can reveal more detail beyond the 1-centimeter resolution captured by the radar. To explain how it works, we'll recall a couple of concepts from high school physics. When out-of-phase waves from the two antennas are combined, constructive and destructive interference patterns result in some wave crests being higher and some wave troughs being lower than those of the original waves. The patterns that result from the combination of the waves reveal more detail with resolution better than the 1-centimeter wavelength of the original Ka-band radar waves because the interference occurs over a portion of a wavelength. An interferogram can be coupled with elevation data to reveal a 3D representation of the water’s surface.
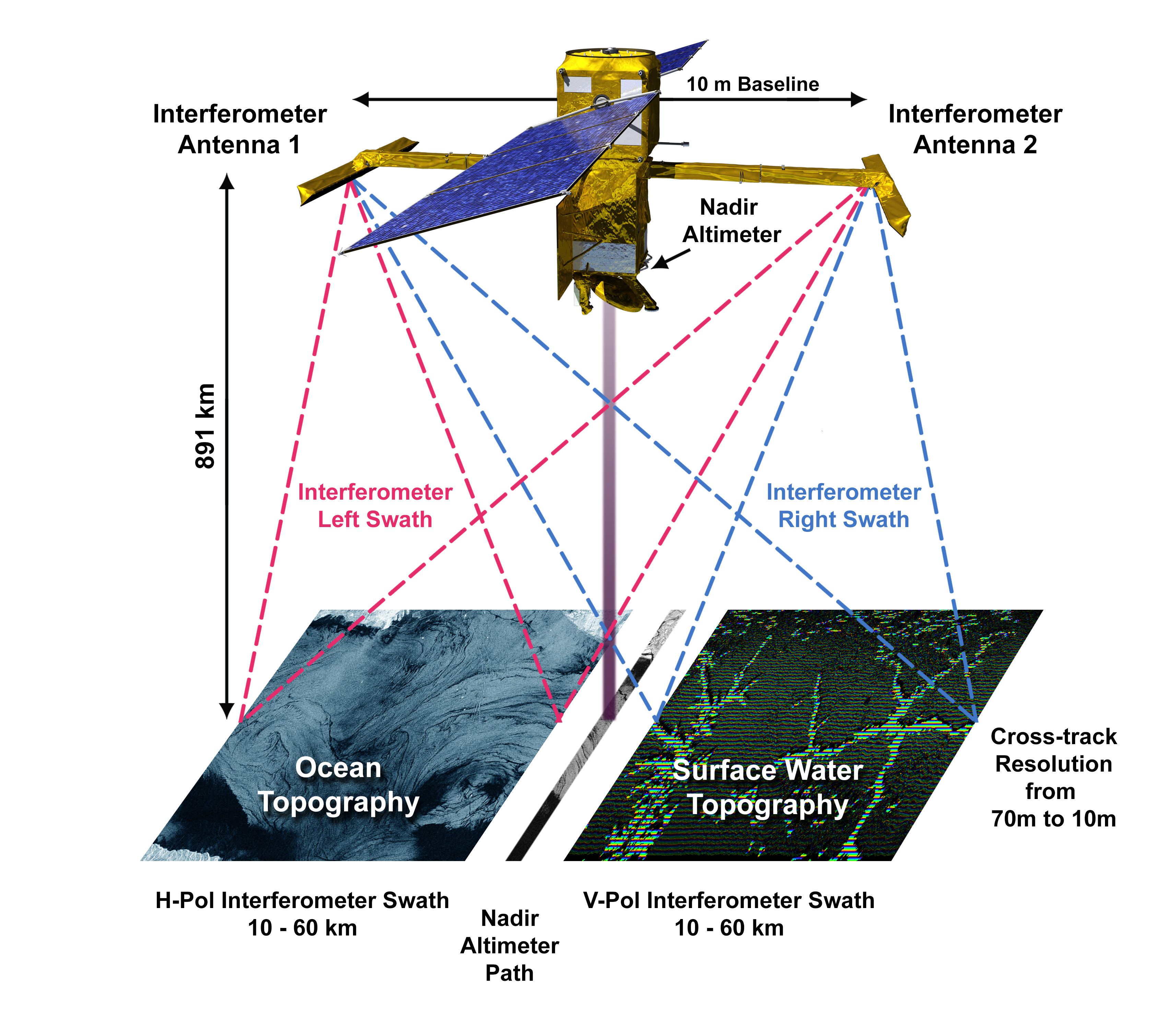
The KaRIn instrument illuminates two parallel tracks of approximately 50 kilometres on either side of a nadir track from a traditional altimeter. The signals are received by two antennas 10 metres apart and are then processed to yield interferometry measurements. Image credit: NASA/JPL-Caltech | + Expand image
This highly accurate 3D view of Earth’s surface water is what makes SWOT so unique and will enable scientists to more closely monitor the dynamics of the water cycle. In addition to observing ocean currents and eddies that will inform our understanding of the ocean’s role in climate change, SWOT's use of interferometry will allow scientists to track volumetric changes in lakes and quantify river flooding, tasks that cannot yet be done on a wide scale in any other way.
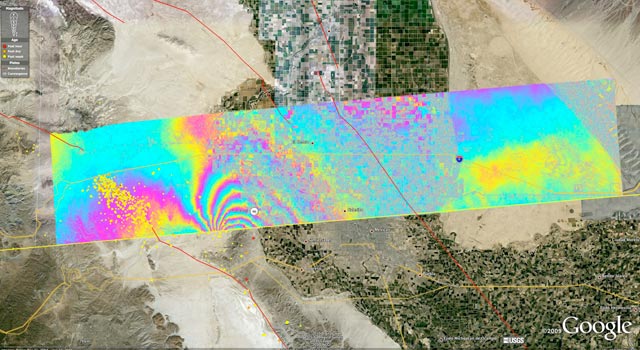
This interferogram was captured by the air-based UAVSAR instrument of the magnitude 7.2 Baja California earthquake of April 4, 2010. The interferogram is overlaid atop a Google Earth image of the region. Image credit: NASA/JPL/USGS/Google | › Learn more
Follow Along
SWOT is scheduled to launch no earlier than Dec. 16, 2022, on a SpaceX Falcon 9 rocket from Vandenberg Space Force Base in California. Tune in to watch the launch on NASA TV.
After launch, the spacecraft will spend 6-months in a calibration and validation phase, during which it will make a full orbit of Earth every day at an altitude of 553 miles (857 kilometers). Upon completion of this phase, SWOT will increase its altitude to 554 miles (891 kilometers) and assume a 21-day repeat orbit for the remainder of its mission.
Visit the mission website to follow along as data are returned and explore the latest news, images, and updates as SWOT provides a new view on one of our planet's most important resources.
Teach It
The SWOT mission is the perfect opportunity to engage students in studying Earth’s water budget and water cycle. Explore these lessons and resources to get students excited about the STEM involved in studying Earth’s water and climate change from space.
Educator Resources
- Collection
SWOT Mission Lessons for Educators
Explore the science and engineering behind the SWOT mission with this collection of standards-aligned lessons all about water.
- Collection
Climate Change Lessons for Educators
Explore a collection of standards-aligned STEM lessons for students that get them investigating climate change along with NASA.
- Collection
Teachable Moments in Climate Change
Explore this collection of Teachable Moments articles to get a primer on the latest NASA Earth science missions, plus find related education resources you can deploy right away!
- Expert Talk
Teaching Space With NASA – Monitoring Earth from Space
In this educational talk, NASA experts discuss how we build spacecraft to study climate, then answer audience questions.
Student Activities
- Collection
SWOT Mission Activities for Students
Explore projects, videos, slideshows, and games for students all about the water cycle and sea level rise.
- Collection
Climate Change Activities for Students
Learn about climate change and its impacts with these projects, videos, and slideshows for students.
- Collection
Earth Minute Video Series
This series of animated white-board videos for students of all ages explains key concepts about Earth science, missions, and climate change.
Explore More
Activities for Kids
- Download: SWOT Launch Bingo
- Video: How Much Water is on Earth?
- Game: Go With the Flow – An Ocean Currents Game
Websites
- SWOT Mission Website
- NASA Climate Change
- NASA Earth Observatory
- NASA Climate Kids
- NASA Sea Level Change
- NASA Cambio Climático en Español
Facts & Figures
Videos
Interactives
Image Gallery
Articles
- Climate articles from NASA
- Ask NASA Climate
- NASA People - Earth
- Water Mission to Gauge Alaskan Rivers on Front Lines of Climate Change
Podcast
TAGS: K-12 Education, Teachers, Educators, Earth Science, Earth, Climate Change, Climate, Satellites, Teachable Moments, Climate TM