Teachable Moments | October 24, 2022
X-Ray Vision and Polarized Glasses Unite to Uncover Mysteries of the Universe
A NASA space telescope mission is giving astronomers a whole new way to peer into the universe, allowing us to uncover long-standing mysteries surrounding objects such as black holes. Find out how it works and how to engage students in the science behind the mission.
Some of the wildest, most exciting features of our universe – from black holes to neutron stars – remain mysteries to us. What we do know is that because of their extreme environments, some of these emit highly energetic X-ray light, which we can detect despite the vast distances between us and the source.
Now, a NASA space telescope mission is using new techniques to not only scout out these distant phenomena, but also provide new information about their origins. Read on to learn how scientists are getting exciting new perspectives on our universe and what the future of X-ray astronomy holds.
How They Did It
In 2021, NASA launched the Imaging X-Ray Polarimeter Explorer, or IXPE, through a collaboration with Ball Aerospace and the Italian Space Agency. The space telescope is designed to operate for two years, detecting X-rays emitted from highly energetic objects in space, such as black holes, different types of neutron stars (e.g., pulsars and magnetars) and active galactic nuclei. In its first year, the telescope is focusing on roughly a dozen previously studied X-ray sources, spending hours or even days observing each target to reveal new data made possible by spacecraft's scientific instruments.
IXPE isn't the first telescope to observe the universe in X-ray light. NASA's Chandra X-ray Observatory, launched in 1999, has famously spent more than 20 years photographing our universe at a wavelength of light exclusively found in high-energy environments, such as where cosmic materials are heated to millions of degrees as a result of intense magnetic fields or extreme gravity.
Using Chandra, scientists can assign colors to the different energy levels, or wavelengths, produced by these environments. This allows us to get a picture of the highly energetic light ejected by black holes and tiny neutron stars – small, but extremely dense stars with masses 10-25 times that of our Sun. These beautiful images, such as from Chandra’s first target, Cassiopeia A (Cas A for short), show the violent beauty of stars exploding.
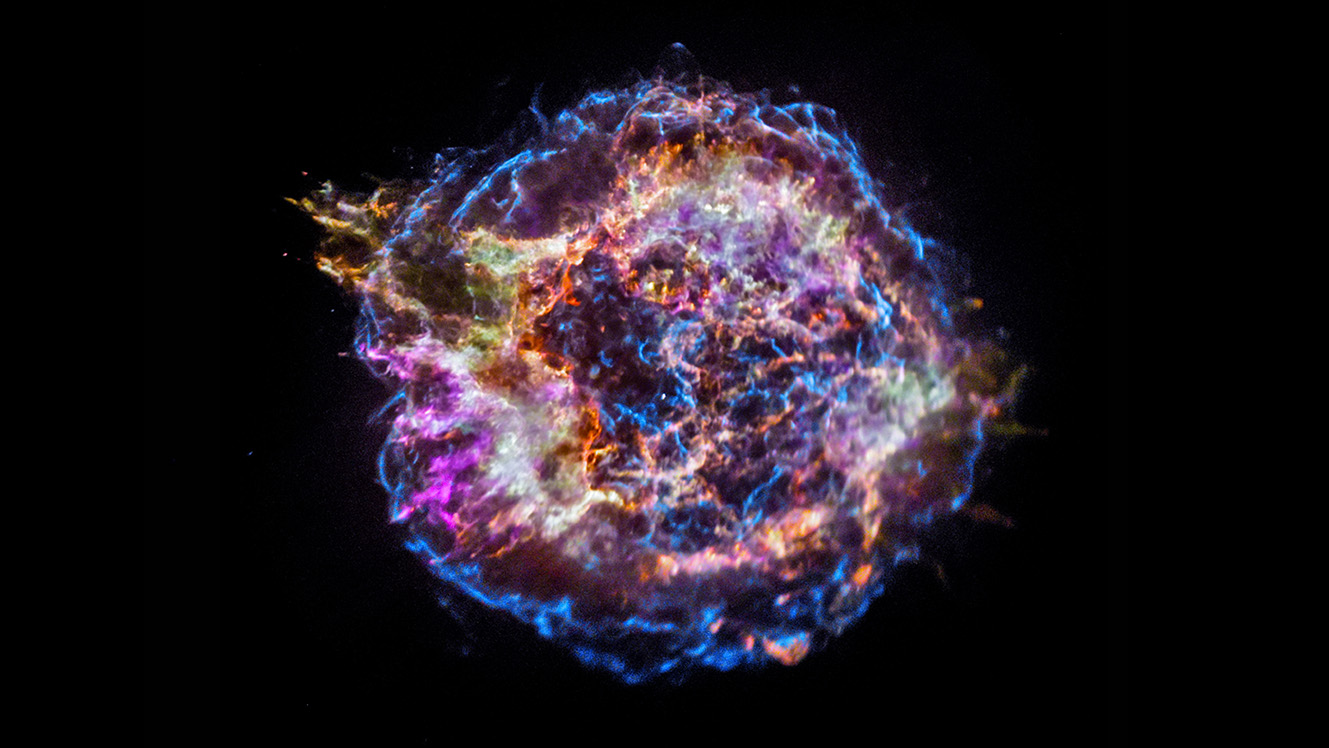
This image of the supernova Cassiopeia A from NASA’s Chandra X-ray Observatory shows the location of different elements in the remains of the explosion: silicon (red), sulfur (yellow), calcium (green) and iron (purple). Each of these elements produces X-rays within narrow energy ranges, allowing maps of their location to be created. Image credit: NASA/CXC/SAO | › Full image and caption
While Chandra has earned its name as one of “The Great Observatories,” astronomers have long desired to peer further into highly energetic environments in space by capturing them in even more detail.
IXPE expands upon Chandra’s work with the introduction of a tool called a polarimeter, an instrument used to understand the shape and direction of the light that reaches the space telescope's detectors. The polarimeter on IXPE allows scientists to gain insight into the finer details of black holes, supernovas, and magnetars, like which direction they are spinning and their three-dimensional shape.
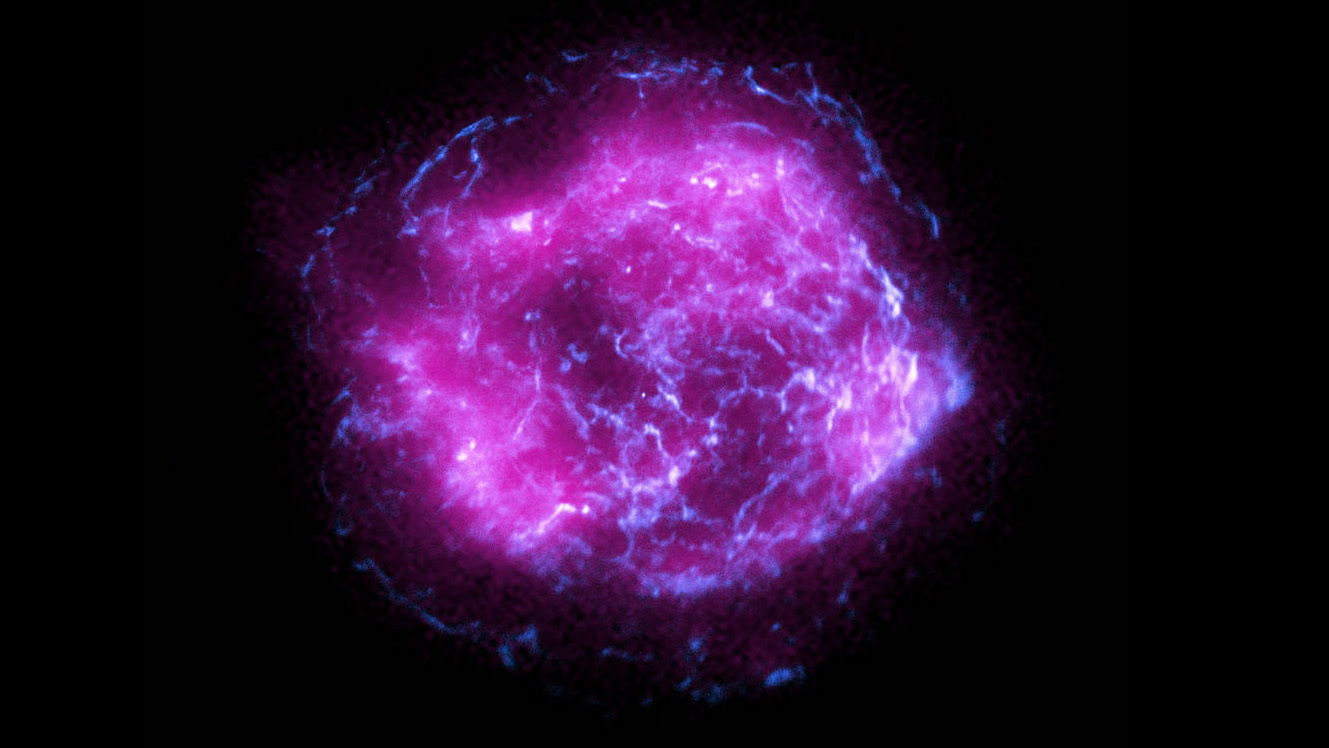
This image of Cassiopeia A was created using some of the first X-ray data collected by IXPE, shown in magenta, combined with high-energy X-ray data from Chandra, in blue. Image credit: NASA/CXC/SAO/IXPE | › Full image and caption
While scientists have just begun putting IXPE's capabilities to use, they're already starting to reveal new details about the inner workings of these objects – such as the magnetic field environment around Cas A, shown in a newly released image.
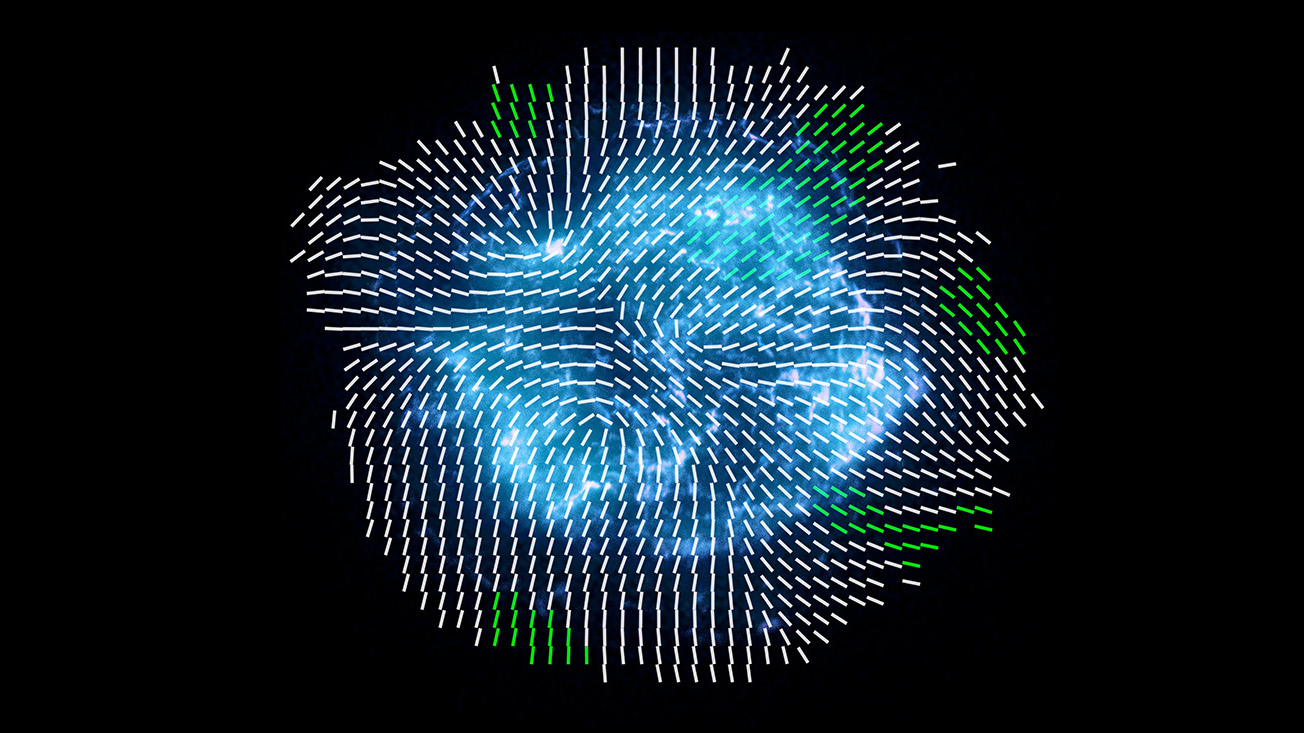
The lines in this newly released image come from IXPE measurements that show the direction of the magnetic field across regions of Cassiopeia A. Green lines indicate regions where the measurements are most highly significant. These results indicate that the magnetic field lines near the outskirts of the supernova remnant are largely oriented radially, i.e., in a direction from the center of the remnant outwards. The IXPE observations also reveal that the magnetic field over small regions is highly tangled, without a dominant preferred direction. Observations such as this one can help scientists learn how particles shooting out from supernovae interact with the magnetic field created by the explosion. Image credits: X-ray: Chandra: NASA/CXC/SAO; IXPE: NASA/MSFC/J. Vink et al. | + Expand image | › Full image and caption
“For the first time, we will use every collected photon of light to tell us about the nature and shapes of objects in the sky that would be dots of light otherwise,” says Roger Romani, a Stanford professor and the co-investigator on IXPE.
How It Works
Generally, when light is produced, it is what we call unpolarized, meaning that it oscillates in every direction. For example, our Sun produces unpolarized light. But sometimes, light is produced in a highly organized fashion, oscillating only in one direction. In astronomy, this arises when magnetic fields force particles to incredibly high speeds, creating highly organized, or polarized, light.
This is what makes objects like the supernova Cas A such enticing targets for IXPE. Exploded stars like Cas A generate massive energetic waves when they go supernova, giving scientists a view of how particles shooting out at immense speeds interact with the magnetic fields from such an event. In the case of Cas A, IXPE was able to determine that the x-rays are not very polarized, meaning the explosion created very turbulent regions with multiple field directions.
While the idea of polarized or organized light may sound abstract, you may have noticed it the last time you were outside on a sunny day. If you’ve tried on a pair of polarized sunglasses, you may have noticed that the glare was greatly reduced. That’s because as light scatters, it bounces off of reflective surfaces in all directions. However, polarized lenses have tiny filters that only allow light coming from a narrow band of directions to pass through.
The polarimeter on IXPE works in a similar way. Astronomers can determine the strength of an object's magnetic field by using the polarimeter to measure how much of the light detected by the telescope is polarized. Typically, the more polarized the light the stronger the magnetic field at the source.
Astronomers can even go a step further to measure the direction this light is oscillating by measuring the angle of the light that reaches the telescope. Because the polarized light leaves the source in a predictable fashion – namely perpendicular from its magnetic field – knowing the angle of the oscillating light provides information about the axis of rotation and potentially even the surface structure of objects such as neutron stars and nebulae.

In this demonstration, the rope represents light waves and the open window represents a polarimeter. Depending on the angle of the light waves (rope), more or less information makes it through the polarimeter (window) the narrower it is. By measuring the amount of light received through the polarimeter, IXPE can determine the angle and the polarization of the light. Image credit: NASA/JPL-Caltech | + Expand image
Imagine, for example, that you were holding one end of a piece of rope secured to an object at the other end. If you swung the rope side to side to make horizontal waves, those waves would be able to make it through a narrow target like a window. If you started to shut the window from the top, narrowing the opening, the waves could conceivably still make it through the opening. However, if you made veritcal waves by waving the rope up and down, as the window closed, fewer and fewer waves would make it through the opening. Likewise, by measuring the light that makes it through the polarimeter to the detector on the other side, IXPE can determine the angle of the light received.
To collect this light, IXPE uses three identical mirrors at the end of a four meter (13 foot) boom. The light received by IXPE is carefully focused on the spacecraft’s polarimeter at the other end of the boom, allowing scientists to collect those crucial measurements.
During the IXPE launch broadcast, commentators discuss the components of the spacecraft and how it measures polarization. | Watch on YouTube
Why It's Important
Building on Chandra's observations from the past two decades, IXPE's novel approach to X-ray science is pulling the curtain back even farther on some of the most fascinating objects in the universe, providing first looks at how and where radiation is being produced in some of the most extreme environments in the universe. IXPE's measurements of Cas A are just the beginning, with even more mysterious targets ready to be explored.
Take it from Martin Weisskopf, the principal scientist on IXPE and project scientist for Chandra, who has spent his 50-year career working in X-ray astronomy, who says, “IXPE will open up the field in ways we’ve been stuck only theorizing about."
Teach It
Explore more on how NASA uses light to map our universe, and dig deeper into some of the celestial features it allows to study, such as blackholes and neutron stars.
Activities
- Project
Exploring Materials - Polarizers
In this activity, learners experiment how polarizers affect light by using two polarizing sheets and overlapping layers of transparent tape.
- Activity Guidebook
Girls STEAM Ahead with NASA Program Cookbook
A guidebook for facilitators planning their own Girls STEAM Ahead with NASA event using NASA’s Universe of Learning resources.
- Slideshow
Black Holes: By the Numbers
What are black holes and how do they form? Explore more in this slideshow.
- Video
What Is a Black Hole?
Find out how what a black hole is, how they can form and why they are so cool!
Educator Resources
-
Science Briefing: Exploring the High Energy Universe
Explore the event materials from this science briefing to get a window into the most energetic processes and the most extreme objects in the universe.
Subject Science
-
The Science of Color
Quickly and easily model how colors reflect, absorb, and interact with each other in the classroom or online using your computer’s camera.
Subject Science
Grades 2-8
Time < 30 mins
-
Using Light to Study Planets
Students build a spectrometer using basic materials as a model for how NASA uses spectroscopy to determine the nature of elements found on Earth and other planets.
Subject Science
Grades 6-11
Time 2+ hrs
-
Dropping In With Gravitational Waves
Students develop a model to represent the collision of two black holes, the gravitational waves that result and the waves' propagation through spacetime.
Subject Science
Grades 6-8
Time 30-60 mins
- Teachable Moments
Telescopes Get Extraordinary View of Milky Way's Black Hole
Find out how scientists captured the first image of Sagittarius A*, why it's important, and how to turn it into a learning opportunity for students.
- Teachable Moments
How Scientists Captured the First Image of a Black Hole
Find out how scientists created a virtual telescope as large as Earth itself to capture the first image of a black hole's silhouette.
- Teachable Moments
Gravitational Waves Detected for the First Time
Find out how researchers proved part of Albert Einstein’s Theory of General Relativity, then create a model of the Nobel Prize-winning experiment in the classroom.
Explore More
- Article: NASA’S IXPE Helps Unlock the Secrets of Famous Exploded Star
- Educator Guide: Black Hole Math
- Opportunity: NASA/IPAC TeacherArchive Research Program
- Student Resources: Chandra
- Article: Hubble - Black Holes
- Interactive: Sagittarius A*
- Video: Hubble - Black Holes
- Website: NASA Science - Black Holes
- Download: A Galaxy Full of Black Holes Presentation
- Expert Talk: Imaging a Black Hole Lecture
- Facts & Figures: NASA - Black Holes
- Article: Black Hole Image Makes History
- Infographic: Anatomy of a Black Hole
NASA's Universe of Learning materials are based upon work supported by NASA under award number NNX16AC65A to the Space Telescope Science Institute, working in partnership with Caltech/IPAC, Center for Astrophysics | Harvard & Smithsonian, and the Jet Propulsion Laboratory.
TAGS: Universe, Stars and Galaxies, Space Telescope, IXPE, Astronomy, Science, Electromagnetic Spectrum, Universe of Learning